CHAPTER V. PalAEontology. |
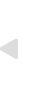 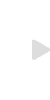 The present Chapter will be devoted to a consideration of the evidence of organic evolution which has been furnished by the researches of geologists. On account of its direct or historical nature, this branch of evidence is popularly regarded as the most important—so much so, indeed, that in the opinion of most educated persons the whole doctrine of organic evolution must stand or fall according to the so-called “testimony of the rocks.” Now, without at all denying the peculiar importance of this line of evidence, I must begin by remarking that it does not present the denominating importance which popular judgment assigns to it. For although popular judgment is right in regarding the testimony of the rocks as of the nature of a history, this judgment, as a rule, is very inadequately acquainted with the great imperfections of that history. Knowing in a general way what magnificent advances the science of geology has made during the present century, the public mind is more or less imbued with the notion, that because we now possess a tolerably complete record of the chronological succession of geological formations, we must therefore possess a correspondingly complete record of the chronological succession of the forms of life which from time to time have peopled the globe. Now in one sense this notion is partly true, but in another sense it is profoundly false. It is partly true if we have regard only to those larger divisions of the vegetable or animal kingdoms which naturalists designate by the terms classes and orders. But the notion becomes progressively more untrue when it is applied to families and genera, while it is most of all untrue when applied to species. That this must be so may be rendered apparent by two considerations. In the first place, it does not follow that because we have a tolerably complete record of the succession of geological formations, we have therefore any correspondingly complete record of their fossiliferous contents. The work of determining the relative ages of the rocks does not require that every cubic mile of the earth’s surface should be separately examined, in order to find all the different fossils which it may contain. Were this the case, we should hitherto have made but very small progress in our reading of the testimony of the rocks. The relative ages of the rocks are determined by broad comparative surveys over extensive areas; and although the identification of widely separated deposits is often greatly assisted by a study of their fossiliferous contents, the mere pricking of a continent here and there is all that is required for this purpose. Hence, the accuracy of our information touching the relative ages of geological strata does not depend upon—and, therefore, does not betoken—any equivalent accuracy of knowledge touching the fossiliferous material which these strata may at the present time actually contain. And, as we well know, the opportunities which the geologist has of discovering fossils are extremely limited, if we consider these opportunities in relation to the area of geological formations. The larger portion of the earth’s surface is buried beneath the sea; and much the larger portion of the fossiliferous deposits on shore are no less hopelessly buried beneath the land. Therefore it is only upon the fractional portion of the earth’s surface which at the present time happens to be actually exposed to his view that the geologist is able to prosecute his search for fossils. But even here how miserably inadequate this search has hitherto been! With the exception of a scratch or two in the continents of Asia and America, together with a somewhat larger number of similar scratches over the continent of Europe, even that comparatively small portion of the earth’s surface which is available for the purpose has been hitherto quite unexplored by the palÆontologist. How enormously rich a store of material remains to be unearthed by the future scratchings of this surface, we may dimly surmise from the astonishing world of bygone life which is now being revealed in the newly discovered fossiliferous deposits on the continent of America. But, besides all this, we must remember, in the second place, that all the fossiliferous deposits in the world, even if they could be thoroughly explored, would still prove highly imperfect, considered as a history of extinct forms of life. In order that many of these forms should have been preserved as fossils, it is necessary that they should have died upon a surface neither too hard nor too soft to admit of their leaving an impression; that this surface should afterwards have hardened sufficiently to retain the impression; that it should then have been protected from the erosion of water, as well as from the disintegrating influence of the air; and yet that it should not have sunk far enough beneath the surface to have come within the no less disintegrating influence of subterranean heat. Remembering thus, as a general rule, how many conditions require to have met before a fossil can have been both formed and preserved, we must conclude that the geological record is probably as imperfect in itself as are our opportunities of reading even the little that has been recorded. If we speak of it as a history of the succession of life upon the planet, we must allow, on the one hand, that it is a history which merits the name of a “chapter of accidents"; and, on the other hand, that during the whole course of its compilation pages were being destroyed as fast as others were being formed, while even of those that remain it is only a word, a line, or at most a short paragraph here and there, that we are permitted to see. With so fragmentary a record as this to study, I do not think it is too much to say that no conclusions can be fairly based upon it, merely from the absence of testimony. Only if the testimony were positively opposed to the theory of descent, could any argument be fairly raised against that theory on the grounds of this testimony. In other words, if any of the fossils hitherto discovered prove the order of succession to have been incompatible with the theory of genetic descent, then the record may fairly be adduced in argument, because we should then be in possession of definite information of a positive kind, instead of a mere absence of information of any kind. But if the adverse argument reaches only to the extent of maintaining that the geological record does not furnish us with so complete a series of “connecting links” as we might have expected, then, I think, the argument is futile. Even in the case of human histories, written with the intentional purpose of conveying information, it is an unsafe thing to infer the non-occurrence of an event from a mere silence of the historian—and this especially in matters of comparatively small detail, such as would correspond (in the present analogy) to the occurrence of species and genera as connecting links. And, of course, if the history had only come down to us in fragments, no one would attach any importance at all to what might have been only the apparent silence of the historian. In view, then, of the unfortunate imperfection of the geological record per se, as well as of the no less unfortunate limitation of our means of reading even so much of the record as has come down to us, I conclude that this record can only be fairly used in two ways. It may fairly be examined for positive testimony against the theory of descent, or for proof of the presence of organic remains of a high order of development in a low level of strata. And it may be fairly examined for negative testimony, or for the absence of connecting links, if the search be confined to the larger taxonomic divisions of the fauna and flora of the world. The more minute these divisions, the more restricted must have been the areas of their origin, and hence the less likelihood of their having been preserved in the fossil state, or of our finding them even if they have been. Therefore, if the theory of evolution is true, we ought not to expect from the geological record a full history of specific changes in any but at most a comparatively small number of instances, where local circumstances happen to have been favourable for the writing and preservation of such a history. But we might reasonably expect to find a general concurrence of geological testimony to the larger fact—namely, of there having been throughout all geological time a uniform progression as regards the larger taxonomic divisions. And, as I will next proceed to show, this is, in a general way, what we do find, although not altogether without some important exceptions, with which I shall deal in an Appendix. There is no positive proof against the theory of descent to be drawn from a study of palÆontology, or proof of the presence of any kind of fossils in strata where the fact of their presence is incompatible with the theory of evolution. On the other hand, there is an enormous body of uniform evidence to prove two general facts of the highest importance in the present connexion. The first of these general facts is, that an increase in the diversity of types both of plants and animals has been constant and progressive from the earliest to the latest times, as we should anticipate that it must have been on the theory of descent in ever-ramifying lines of pedigree. And the second general fact is, that through all these branching lines of ever-multiplying types, from the first appearance of each of them to their latest known conditions, there is overwhelming evidence of one great law of organic nature—the law of gradual advance from the general to the special, from the low to the high, from the simple to the complex. Now, the importance of these large and general facts in the present connexion must be at once apparent; but it may perhaps be rendered more so if we try to imagine how the case would have stood supposing geological investigation to have yielded in this matter an opposite result, or even so much as an equivocal result. If it had yielded an opposite result, if the lower geological formations were found to contain as many, as diverse, and as highly organized types as the later geological formations, clearly there would have been no room at all for any theory of progressive evolution. And, by parity of reasoning, in whatever degree such a state of matters were found to prevail, in that degree would the theory in question have been discredited. But seeing that these opposite principles do not prevail in any (relatively speaking) considerable degree[16], we have so far positive testimony of the largest and most massive character in favour of this theory. For while all these large and general facts are very much what they ought to be according to this theory, they cannot be held to lend any support at all to the rival theory. In other words, it is clearly no essential part of the theory of special creation that species should everywhere exhibit this gradual multiplication as to number, coupled with a gradual diversification and general elevation of types, in all the growing branches of the tree of life. No one could adopt seriously the jocular lines of Burns, to the effect that the Creator required to practise his prentice hand on lower types before advancing to the formation of higher. Yet, without some such assumption, it would be impossible to explain, on the theory of independent creations, why there should have been this gradual advance from the few to the many, from the general to the special, from the low to the high. I submit, then, that so far as the largest and most general principles in the matter of palÆontology are concerned, we have about as strong and massive a body of evidence as we could reasonably expect this branch of science to yield; for it is at once enormous in amount and positive in character. Therefore, if I do not further enlarge upon the evidence which we here have, as it were en masse, it is only because I do not feel that any words could add to its obvious significance. It may best be allowed to speak for itself in the millions of facts which are condensed in this tabular statement of the order of succession of all the known forms of animal life, as presented by the eminent palÆontologist, Professor Cope[17]. Or, taking a still more general survey, this tabular statement may be still further condensed, and presented in a diagrammatic form, as it has been by another eminent American palÆontologist, Prof. Le Conte, in his excellent little treatise on Evolution and its Relations to Religious Thought. The following is his diagrammatic representation, with his remarks thereon. When each ruling class declined in importance, it did not perish, but continued in a subordinate position. Thus, the whole organic kingdom became not only higher and higher in its highest forms, but also more and more complex in its structure and in the interaction of its correlated parts. The whole process and its result is roughly represented in the accompanying diagram, in which A B represents the course of geological time, and the curve, the rise, culmination, and decline of successive dominate classes. Geological Succession of the Classes of the Animal Kingdom. Fig. 59.—Diagram of Geological Succession of the Classes of the Animal Kingdom. (After Le Conte.) I will here leave the evidence which is thus yielded by the most general principles that have been established by the science of palÆontology; and I will devote the rest of this chapter to a detailed consideration of a few highly special lines of evidence. By thus suddenly passing from one extreme to the other, I hope to convey the best idea that can be conveyed within a brief compass of the minuteness, as well as the extent, of the testimony which is furnished by the rocks. When Darwin first published his Origin of Species, adverse critics fastened upon the “missing-link” argument as the strongest that they could bring against the theory of descent. Although Darwin had himself strongly insisted on the imperfection of the geological record, and the consequent precariousness of any negative conclusions raised upon it, these critics maintained that he was making too great a demand upon the argument from ignorance—that, even allowing for the imperfection of the record, they would certainly have expected at least a few cases of testimony to specific transmutation. For, they urged in effect, looking to the enormous profusion of the extinct species on the one hand, and to the immense number of known fossils on the other, it was incredible that no satisfactory instances of specific transmutation should ever have been brought to light, if such transmutation had ever occurred in the universal manner which the theory was bound to suppose. But since Darwin first published his great work palÆontologists have been very active in discovering and exploring fossiliferous beds in sundry parts of the world; and the result of their labours has been to supply so many of the previously missing links that the voice of competent criticism in this matter has now been well-nigh silenced. Indeed, the material thus furnished to an advocate of evolution at the present time is so abundant that his principal difficulty is to select his samples. I think, however, that the most satisfactory result will be gained if I restrict my exposition to a minute account of some few series of connecting links, rather than if I were to take a more general survey of a larger number. I will, therefore, confine the survey to the animal kingdom, and there mention only some of the cases which have yielded well-detailed proof of continuous differentiation. It is obvious that the parts of animals most likely to have been preserved in such a continuous series of fossils as the present line of evidence requires, would have been the hard parts. These are horns, bones, teeth, and shells. Therefore I will consider each of these four classes of structures separately. Horns wherever they occur, are found to be of high importance for purposes of classification. They are restricted to the Ruminants, and appear under three different forms or types—namely solid, as in antelopes; hollow, as in sheep; and deciduous, as in deer. Now, in each of these divisions we have a tolerably complete palÆontological history of the evolution of horns. The early ruminants were altogether hornless (Fig. 60). Then, in the middle Miocene, the first antelopes appeared with tiny horns, which progressively increased in size among the ever-multiplying species of antelopes until the present day. But it is in the deer tribe that we meet with even better evidence touching the progressive evolution of horns; because here not only size, but shape, is concerned. For deer’s horns, or antlers, are arborescent; and hence in their case we have an opportunity of reading the history, not only of a progressive growth in size, but also of an increasing development of form. Among the older members of the tribe, in the lower Miocene, there are no horns at all. In the mid-Miocene we meet with two-pronged horns (Cervus dicrocerus, Figs. 61, 62, 1/5 nat. size). Next, in the upper Miocene (C. matheronis, Fig. 63, 1/8 nat. size), and extending into the Pliocene (C. pardinensis, Fig. 64, 1/18 nat. size), we meet with three-pronged horns. Then, in the Pliocene we find also four-pronged horns (C. issiodorensis, Fig. 65, 1/16 nat. size), leading us to five-pronged (C. tetraceros). Lastly, in the Forest-bed of Norfolk we meet with arborescent horns (C. Sedgwickii, Fig. 66, 1/35 nat. size). The life-history of existing stags furnishes a parallel development (Fig. 67), beginning with a single horn (which has not yet been found palÆontologically), going on to two prongs, three prongs, four prongs, and afterwards branching. Fig. 60.—Skull of Oreodon Culbertsoni. (After Leidy.) Fig. 60.—Skull of Oreodon Culbertsoni. (After Leidy.) Evolution of horns. Figs. 61-6. The series is reduced from Gaudry’s illustrations, after Farge, Croizet, Jobert and Boyd Dawkins. Successive stages in the development of an existing Deer’s Antlers. Fig. 67. Successive stages in the development of an existing Deer’s Antlers. (After Gaudry, but a better illustration has already been given on p. 100.) Coming now to bones, we have a singularly complete record of transition from one type or pattern of structure to another in the phylogenetic history of tails. This has been so clearly and so tersely conveyed by Prof. Le Conte, that I cannot do better than quote his statement. It has long been noticed that there are among fishes two styles of tail-fins. These are the even-lobed, or homocercal (Fig. 68), and the uneven-lobed, or heterocercal (Fig. 69). The one is characteristic of ordinary fishes (teleosts), the other of sharks and some other orders. In structure the difference is even more fundamental than in form. In the former style the backbone stops abruptly in a series of short, enlarged joints, and thence sends off rays to form the tail-fin (Fig. 68); in the latter the backbone runs through the fin to its very point, growing slenderer by degrees, and giving off rays above and below from each joint, but the rays on the lower side are much longer (Fig. 69). This type of fin is, therefore, vertebrated, the other non-vertebrated. Figs. 68 and 69 show these two types in form and structure. But there is still another type found only in the lowest and most generalized forms of fishes. In these the tail-fin is vertebrated and yet symmetrical. This type is shown in Fig. 70. Homocercal Tail. Fig. 68.—Homocercal Tail, showing (A) external form and (B) internal structure. Heterocercal Tail. Fig. 69.—Heterocercal Tail, showing (A) external form and (B) internal structure. Diphycercal fin. Fig. 70.—Vertebrated but symmetrical fin (diphycercal), showing (A) external form and (B) internal structure. Now, in the development of a teleost fish (Fig. 68), as has been shown by Alexander Agassiz, the tail-fin is first like Fig. 70; then becomes heterocercal, like Fig. 69; and, finally, becomes homocercal like Fig. 68. Why so? Not because there is any special advantage in this succession of forms; for the changes take place either in the egg or else in very early embryonic states. The answer is found in the fact that this is the order of change in the phylogenetic series. The earliest fish-tails were either like Fig. 69 or Fig. 70; never like Fig. 68. The earliest of all were almost certainly like Fig. 70; then they became like Fig. 69; and, finally, only much later in geological history (Jurassic or Cretaceous), they became like Fig. 68. This order of change is still retained in the embryonic development of the last introduced and most specialized order of existing fishes. The family history is repeated in the individual history. Fig. 71.—Tail of ArchÆopteryx. Fig. 71.—Tail of ArchÆopteryx. A indicates origin of simply-jointed tail. | Fig. 72.—Tail of modern Bird. Fig. 72.—Tail of modern Bird. The numerals indicate the foreshortened, enlarged, and consolidated joints; f, terminal segment of the vertebral column; D, shafts of feathers. | Fig. 73.—ArchÆopteryx macura, restored. Fig. 73.—ArchÆopteryx macura, restored, ½ nat. size. (After Flower.) The section of the tail is copied from Owen, nat. size. Similar changes have taken place in the form and structure of birds’ tails. The earliest bird known—the Jurassic ArchÆopteryx—had a long reptilian tail of twenty-one joints, each joint bearing a feather on each side, right and left (Fig. 71): [see also Fig. 73]. In the typical modern bird, on the contrary, the tail-joints are diminished in number, shortened up, and enlarged, and give out long feathers, fan-like, to form the so-called tail (Fig. 72). The ArchÆopteryx’ tail is vertebrated, the typical bird’s non-vertebrated. This shortening up of the tail did not take place at once, but gradually. The Cretaceous birds, intermediate in time, had tails intermediate in structure. The Hesperornis of Marsh had twelve joints. At first—in Jurassic strata—the tail is fully a half of the whole vertebral column. It then gradually shortens up until it becomes the aborted organ of typical modern birds. Now, in embryonic development, the tail of the modern typical bird passes through all these stages. At first the tail is nearly one half the whole vertebral column; then, as development goes on, while the rest of the body grows, the growth of the tail stops, and thus finally becomes the aborted organ we now find. The ontogeny still passes through the stages of the phylogeny. The same is true of all tailless animals. The extinct ArchÆopteryx above alluded to presents throughout its whole organization a most interesting assemblage of “generalized characters.” For example, its teeth, and its still unreduced digits of the wings (which, like those of the feet, are covered with scales), refer us, with almost as much force as does the vertebrated tail, to the Sauropsidian type—or the trunk from which birds and reptiles have diverged. We will next consider the palÆontological evidence which we now possess of the evolution of mammalian limbs, with special reference to the hoofed animals, where this line of evidence happens to be most complete. I may best begin by describing the bones as these occur in the sundry branches of the mammalian type now living. As we shall presently see, the modifications which the limbs have undergone in these sundry branches chiefly consist in the suppression of some parts and the exaggerated development of others. But, by comparing all mammalian limbs together, it is easy to obtain a generalized type of mammalian limb, which in actual life is perhaps most nearly conformed to in the case of bears. I will therefore choose the bear for the purpose of briefly expounding the bones of mammalian limbs in general—merely asking it to be understood, that although in the case of many other mammalia some of these bones may be dwindled or altogether absent, while others may be greatly exaggerated as to relative size, in no case do any additional bones appear. On looking, then, at the skeleton of a bear (Fig. 74), the first thing to observe is that there is a perfect serial homology between the bones of the hind legs and of the fore legs. The thigh-bone, or femur, corresponds to the shoulder-bone, or humerus; the two shank bones (tibia and fibula) correspond to the two arm-bones (radius and ulna); the many little ankle-bones (tarsals) correspond to the many little wrist-bones (carpals); the foot-bones (meta-tarsals) correspond to the hand-bones (meta-carpals); and, lastly, the bones of each of the toes correspond to those of each of the fingers. Skeleton of Polar Bear. Fig. 74.—Skeleton of Polar Bear, drawn from nature (Brit. Mus.). The next thing to observe is, that the disposition of bones in the case of the bear is such that the animal walks in the way that has been called plantigrade. That is to say, all the bones of the fingers, as well as those of the toes, feet, and ankles, rest upon the ground, or help to constitute the “soles.” Our own feet are constructed on a closely similar pattern. But in the majority of living mammalian forms this is not the case. For the majority of mammals are what has been called digitigrade. That is to say, the bones of the limb are so disposed that both the foot and hand bones, and therefore also the ankle and wrist, are removed from the ground altogether, so that the animal walks exclusively upon its toes and fingers—as in the case of this skeleton (Fig. 75), which is the skeleton of a lion. The next figures display a series of limbs, showing the progressive passage of a completely plantigrade into a highly digitigrade type—the curved lines of connexion serving to indicate the homologous bones (Figs. 76, 77). Skeleton of Lion. Fig. 75.—Skeleton of Lion. (After Huxley.) Anterior limb of Man, Dog, Hog, Sheep, and Horse. Fig. 76.—Anterior limb of Man, Dog, Hog, Sheep, and Horse. (After Le Conte.) Sc, shoulder-blade; c, coracoid; a, b, bones of fore-arm; 5, bones of the wrist; 6, bones of the hand; 7, bones of the fingers. Posterior limb of Man, Monkey, Dog, Sheep and Horse. Fig. 77.—Posterior limb of Man, Monkey, Dog, Sheep and Horse. (After Le Conte.) 1, Hip-joint; 2, thigh-bone; 3, knee-joint; 4, bones of leg; 5, ankle-joint; 6, bones of foot; 7, bones of toes. I will now proceed to detail the history of mammalian limbs, as this has been recorded for us in fossil remains. Posterior limb of Baptanodon discus and anterior limb of Chelydra serpentina. Fig. 78.—A, posterior limb of Baptanodon discus. (After Marsh.) F, thigh-bone; I to VI, undifferentiated bones of the leg and foot. B, anterior limb of Chelydra serpentina. (After Gegenbaur.) U and R, bones of the fore-arm; I to V, fully differentiated bones of the hand, following those of the wrist. The most generalized or primitive types of limb hitherto discovered in any vertebrated animal above the class of fishes, are those which are met with in some of the extinct aquatic reptiles. Here, for instance, is a diagram of the left hind limb of Baptanodon discus (Fig. 78). It has six rows of little symmetrical bones springing from a leg-like origin. Paddle of a Whale. Fig. 79.—Paddle of a Whale. But the whole structure resembles the fin of a fish about as nearly as it does the leg of a mammal. For not only are there six rows of bones, instead of five, suggestive of the numerous rays which characterise the fin of a fish; but the structure as a whole, having been covered over with blubber and skin, was throughout flexible and unjointed—thus in function, even more than in structure, resembling a fin. In this respect, also, it must have resembled the paddle of a whale (see Fig. 79); but of course the great difference will be noted, that the paddle of a whale reveals the dwindled though still clearly typical bones of a true mammalian limb; so that although in outward form and function these two paddles are alike, their inward structure clearly shows that while the one testifies to the absence of evolution, the other testifies to the presence of degeneration. If the paddle of Baptanodon had occurred in a whale, or the paddle of a whale had occurred in Baptanodon, either fact would in itself have been well-nigh destructive of the whole theory of evolution. Such, then, is the most generalized as it is the most ancient type of vertebrate limb above the class of fishes. Obviously it is a type suited only to aquatic life. Consequently, when aquatic Vertebrata began to become terrestrial, the type would have needed modification in order to serve for terrestrial locomotion. In particular, it would have needed to gain in consolidation and in firmness, which means that it would have needed also to become jointed. Accordingly, we find that this archaic type gave place in land-reptiles to the exigencies of these requirements. Here for example is a diagram, copied from Gegenbaur, of the right fore-foot of Chelydra serpentina (Fig. 78). As compared with the homologous limb of its purely aquatic predecessor, there is to be noticed the disappearance of one of the six rows of small bones, a confluence of some of the remainder in the other five rows, a duplication of the arm-bone into a radius and ulna, in order to admit of jointed rotation of the hand, and a general disposition of the small bones below these arm-bones, which clearly foreshadows the joint of the wrist. Indeed, in this fore-foot of Chelydra, a child could trace all the principal homologies of the mammalian counterpart, growing, like the next stage in a dissolving view, out of the primitive paddle of Baptanodon—namely, first the radius and ulna, next the carpals, then the meta-carpals, and, lastly, the three phalanges in each of the five digits. Such a type of foot no doubt admirably meets the requirements of slow reptilian locomotion over swampy ground. But for anything like rapid locomotion over hard and uneven ground, greater modifications would be needed. Such modifications, however, need not be other in kind: it is enough that they should continue in the same line of advance, so as to reach a higher degree of firmness, combined with better joints. Accordingly we find that this took place, not indeed among reptiles, whose habits of cold-blooded life have not changed, but among their warm-blooded descendants, the mammals. Moreover, when we examine the whole mammalian series, we find that the required modifications must have taken place in slightly different ways in three lines of descent simultaneously. We have first the plantigrade and digitigrade modifications already mentioned (pp. 178, 179) Of these the plantigrade walking entailed least change, because most resembling the ancestral or lizard-like mode of progression. All that was here needed was a general improvement as to relative lengths of bones, with greater consolidation and greater flexibility of joints. Therefore I need not say anything more about the plantigrade division. But the digitigrade modification necessitated a change of structural plan, to the extent of raising the wrist and ankle joints off the ground, so as to make the quadruped walk on its fingers and toes. We meet with an interesting case of this transition in the existing hare, which while at rest supports itself on the whole hind foot after the manner of a plantigrade animal, but when running does so upon the ends of its toes, after the manner of a digitigrade animal. It is of importance for us to note that this transition from the original plantigrade to the more recent digitigrade type, has been carried out on two slightly different plans in two different lines of mammalian descent. The hoofed mammals—which are all digitigrade—are sub-classified as artiodactyls and perissodactyls, i. e. even-toed and odd-toed. Now, whether an animal has an even or an odd number of toes may seem a curiously artificial distinction on which to found so important a classification of the mammalian group. But if we look at the matter from a less empirical and more intelligent point of view, we shall see that the alternative of having an even or an odd number of toes carries with it alternative consequences of a practically important kind to any animal of the digitigrade type. For suppose an aboriginal five-toed animal, walking on the ends of its five toes, to be called upon to resign some of his toes. If he is left with an even number, it must be two or four; and in either case the animal would gain the firmest support by so disposing his toes as to admit of the axis of his foot passing between an equal number of them—whether it be one or two toes on each side. On the other hand, if our early mammal were called upon to retain an odd number of toes, he would gain best support by adjusting matters so that the axis of his foot should be coincident with his middle toe, whether this were his only toe, or whether he had one on either side of it. This consideration shows that the classification into even-toed and odd-toed is not so artificial as it no doubt at first sight appears. Let us, then, consider the stages in the evolution of both these types of feet. Going back to the reptile Chelydra, it will be observed that the axis of the foot passes down the middle toe, which is therefore supported by two toes on either side (Fig. 78). It may also be noticed that the wrist or ankle bones do not interlock, either with one another or with the bones of the hand or foot below them. This, of course, would give a weak foot, suited to slow progression over marshy ground—which, as we have seen, was no doubt the origin of the mammalian plantigrade foot. Here, for instance, to all intents and purposes, is a similar type of foot, which belonged to a very early mammal, antecedent to the elephant series, the horse series, the rhinoceros, the hog, and, in short, all the known hoofed mammalia (Fig. 80). It was presumably an inhabitant of swampy ground, slow in its movements, and low in its intelligence. Fossil skeleton of Phenacodus primavus. Fig. 80.—Fossil skeleton of Phenacodus primavus. (After Cope.) But now, as we have seen, for more rapid progression on hard uneven ground, a stronger and better jointed foot would be needed. Therefore we find the bones of the wrist and ankle beginning to interlock, both among themselves and also with those of the foot and hand immediately below them. Such a stage of evolution is still apparent in the now existing elephant. (See Fig. 81.) Bones of the foot of four forms of the perissodactyl type. Fig. 81.—Bones of the foot of four different forms of the perissodactyl type, showing gradual reduction in the number of digits, coupled with a greater consolidation of the bones above the digits. The series reads from right to left. Drawn from nature (Brit. Mus.). Next, however, a still stronger foot was made by the still further interlocking of the wrist and ankle bones, so that both the first and second rows of them were thus fitted into each other, as well as into the bones of the hand and foot beneath. This further modification is clearly traceable in some of the earlier perissodactyls, and occurs in the majority at the present time. Compare, for example, the greater interlocking and consolidation of these small bones in the Rhinoceros as contrasted with the Elephant (Fig. 81). Moreover, simultaneously with these consolidating improvements in the mechanism of the wrist and ankle joints, or possibly at a somewhat later period, a reduction in the number of digits began to take place. This was a continuation of the policy of consolidating the foot, analogous to the dropping out of the sixth row of small bones in the paddle of Baptanodon. (Fig. 78.) In the pentadactyl plantigrade foot of the early mammals, the first digit, being the shortest, was the first to leave the ground, to dwindle, and finally to disappear. More work being thus thrown on the remaining four, they were strengthened by interlocking with the wrist (or ankle) bones above them, as just mentioned; and also by being brought closer together. Bones of the foot of four forms of the artiodactyl type. Fig. 82.—Bones of the foot of four different forms of the artiodactyl type, showing gradual reduction of the number of digits, coupled with a greater consolidation of the bones above the digits. The series reads from right to left. Drawn from nature (Brit. Mus.). The changes which followed I will render in the words of Professor Marsh. Two kinds of reduction began. One leading to the existing perissodactyl foot, and the other, apparently later, resulting in the artiodactyl type. In the former the axis of the foot remained in the middle of the third digit, as in the pentadactyl foot. [See Fig. 81.] In the latter, it shifted to the outer side of this digit, or between the third and fourth toe. [See Fig. 82.] In the further reduction of the perissodactyl foot, the fifth digit, being shorter than the remaining three, next left the ground, and gradually disappeared. [Fig. 81 B.] Of the three remaining toes, the middle or axial one was the longest, and retaining its supremacy as greater strength and speed were required, finally assumed the chief support of the foot [Fig. 81 C], while the outer digits left the ground, ceased to be of use, and were lost, except as splint-bones [Fig. 81 D]. The feet of the existing horse shows the best example of this reduction in the Perissodactyls, as it is the most specialized known in the Ungulates [Fig. 81 D]. In the artiodactyl foot, the reduction resulted in the gradual diminution of the two outer of the four remaining toes, the third and fourth doing all the work, and thus increasing in size and power. The fifth digit, for the same reasons as in the perissodactyl foot, first left the ground and became smaller. Next, the second soon followed, and these two gradually ceased to be functional, [and eventually disappeared altogether, as shown in the accompanying drawing of the feet of still existing animals, Fig. 82 B, C, D]. The limb of the modern race-horse is a nearly perfect piece of machinery, especially adapted to great speed on dry, level ground. The limb of an antelope, or deer, is likewise well fitted for rapid motion on a plain, but the foot itself is adapted to rough mountain work as well, and it is to this advantage, in part, that the Artiodactyls owe their present supremacy. The plantigrade pentadactyl foot of the primitive Ungulate—and even the perissodactyl foot that succeeded it—both belong to the past humid period of the world’s history. As the surface of the earth slowly dried up, in the gradual desiccation still in progress, new types of feet became a necessity, and the horse, antelope, and camel were gradually developed, to meet the altered conditions. The best instance of such progressive modifications in the case of perissodactyl feet is furnished by the fossil pedigree of the existing horse, because here, within the limits of the same continuous family line, we have presented the entire series of modifications. Feet and teeth in fossil pedigree of the Horse. Fig. 83.—Feet and teeth in fossil pedigree of the Horse. (After Marsh.) a, bones of the fore-foot; b, bones of the hind-foot; c, radius and ulna; d, tibia and fibula; e, roots of a tooth; f and g, crowns of upper and lower molar teeth. PalÆotherium. Fig. 84.—PalÆotherium. (Lower Tertiary of Paris Basin.) There are now known over thirty species of horse-like creatures, beginning from the size of a fox, then progressively increasing in bulk, and all standing in linear series in structure as in time. Confining attention to the teeth and feet, it will be seen from the wood-cut on page 189 that the former grow progressively longer in their sockets, and also more complex in the patterns of their crowns. On the other hand, the latter exhibit a gradual diminution of their lateral toes, together with a gradual strengthening of the middle one. (See Fig. 83.) So that in the particular case of the horse-ancestry we have a practically complete chain of what only a few years ago were “missing links.” And this now practically completed chain shows us the entire history of what happens to be the most peculiar, or highly specialized, limb in the whole mammalian class—namely, that of the existing horse. Of the other two wood-cuts, the former (Fig. 84) shows the skeleton of a very early and highly generalized ancestor, while the other is a partial restoration of a much more recent and specialized one. (Fig. 85.) Hipparion. Fig. 85.—Hipparion. (New World Pliocene.) On the other hand, progressive modifications of the artiodactyl feet may be traced geologically up to the different stages presented by living ruminants, in some of which it has proceeded further than in others. For instance, if we compare the pig, the deer, and the camel (Fig. 82), we immediately perceive that the dwindling of the two rudimentary digits has proceeded much further in the case of the deer than in that of the pig, and yet not so far as in that of the camel, seeing that here they have wholly disappeared. Moreover, complementary differences are to be observed in the degree of consolidation presented by the two useful digits. For while in the pig the two foot-bones are still clearly distinguishable throughout their entire length, in the deer, and still more in the camel, their union is more complete, so that they go to constitute a single bone, whose double or compound character is indicated externally only by a slight bifurcation at the base. Nevertheless, if we examine the state of matters in the unborn young of these animals, we find that the two bones in question are still separated throughout their length. and thus precisely resemble what used to be their permanent condition in some of the now fossil species of hoofed mammalia. Turning next from bones of the limb to other parts of the mammalian skeleton, let us briefly consider the evidence of evolution that is here likewise presented by the vertebral column, the skull, and the teeth. As regards the vertebral column, if we examine this structure in any of the existing hoofed animals, we find that the bony processes called zygapophyses, which belong to each of the constituent vertebrÆ, are so arranged that the anterior pair belonging to each vertebra interlocks with the posterior pair belonging to the next vertebra. In this way the whole series of vertebrÆ are connected together in the form of a chain, which, while admitting of considerable movement laterally, is everywhere guarded against dislocation. But if we examine the skeletons of any ungulates from the lower Eocene deposits, we find that in no case is there any such arrangement to secure interlocking. In all the hoofed mammals of this period the zygapophyses are flat. Now, from this flat condition to the present condition of full interlocking we obtain a complete series of connecting links. In the middle Miocene period we find a group of hoofed animals in which the articulation begins by a slight rounding of the previously flat surfaces: later on this rounding progressively increases, until eventually we get the complete interlocking of the present time. As regards teeth, and still confining attention to the hoofed mammals, we find that low down in the geological series the teeth present on their grinding surfaces only three simple tubercles. Later on a fourth tubercle is added, and later still there is developed that complicated system of ridges and furrows which is characteristic of these teeth at the present time, and which was produced by manifold and various involutions of the three or four simple tubercles of Eocene and lower Miocene times. In other words, the principle of gradual improvement in the Construction of teeth, which has already been depicted as regards the particular case of the horse-family (Fig. 83), is no less apparent in the pedigree of all the other mammalia, wherever the palÆontological history is sufficiently intact to serve as a record at all. Comparative series of Brains. Fig. 86.—Comparative series of Brains. (After Le Conte.) The series reads from above downwards, and represents diagrammatically the brain of a Fish, a Reptile, a Bird, a Mammal, and a Man. In each case the letter A marks a side view, and the letter B a top view. The small italics throughout signify the following homologous parts: m, medulla; cb, cerebellum; op, optic lobes; cr, cerebrum and thalamus; ol, olfactory lobes. The series shows a progressive consolidation and enlargement of the brain in general, and of the cerebrum and cerebellum in particular, which likewise exhibit continually advancing structure in respect of convolution. In the case of Man, these two parts of the brain have grown to so great a size that they conceal all the other parts from the superficial points of view represented in the diagram. Ideal section through all the above stages. Fig. 87.—Ideal section through all the above stages. (After Le Conte.) Lastly, as regards the skull, casts of the interior show that all the earlier mammals had small brains with comparatively smooth or unconvoluted surfaces; and that as time went on the mammalian brain gradually advanced in size and complexity. Indeed so small were the cerebral hemispheres of the primitive mammals that they did not overlap the cerebellum, while their smoothness must have been such as in this respect to have resembled the brain of a bird or reptile. This, of course, is just as it ought to be, if the brain, which the skull has to accommodate, has been gradually evolved into larger and larger proportions in respect of its cerebral hemispheres, or the upper masses of it which constitute the seat of intelligence. Thus, if we look at the above series of wood-cuts, which represents the comparative structure of the brain in the existing classes of the Vertebrata, we can immediately understand why the fossil skulls of Mammalia should present a gradual increase in size and furrowing, so as to accommodate the general increase of the brain in both these respects between the level marked “maml” and that marked “man,” in the last of the diagrams. (Fig. 87.) The tabular statement on the following diagram, which I borrow from Prof. Cope, will serve at a glance to reveal the combined significance of so many lines of evidence, united within the limits of the same group of animals. To give only one special illustration of the principle of evolution as regards the skull, here is one of the most recent instances that has occurred of the discovery of a missing link, or connecting form (see Fig. 88). The fossil (B), which was found in New Jersey, stands in an intermediate position between the stag and the elk. In the stag (A) the skull is high, showing but little of that anterior attenuation which is such a distinctive feature of the skull of the elk (C). The nasal bones (N) of the former, again, are remarkably long when compared with the similar bones of the latter, and the premaxillaries (PMX), instead of being projected forward along the horizontal plane of the base of the skull, are deflected sharply downward. In all these points, it will be seen, the newly discovered form (Cervalces) holds an intermediate position (B). “The skull exhibits a partial attenuation anteriorly, the premaxillaries are directed about equally downward and forward, and the nasal bones are measurably contracted in size. The horns likewise furnish characters which further serve to establish this dual relationship[18].” Skulls of Canadian Stag, Cervalces Americanus and Elk. Fig. 88.—Skulls of—A, Canadian Stag; B, Cervalces Americanus; and C, Elk. (After Heilprin.) Formation. | No. of toes. | Feet. | Astragalus. | Carpus and tarsus. | Ulno-radius | Superior molars. | Zygapophyses. | Brain. | Pliocene. | 1-1 2-2 | Digitigrade. (Plantigrade.) | Grooved. (Flat.) | Interlocking. (Opposite.) | Faceted. | 4-tubercles, crested and cemented | Doubly involute. Singly involute. | Hemispheres larger, convoluted. | Upper Miocene. (Loup Fork.) | 3-3 4-4 (5-5) | | | | | | | | Middle. (John Day.) | 2-2 3-3 4-4 | Digitigrade. | Grooved. | Interlocking. | Faceted. Smooth. | 4-tubercles, and crested. | Singly involute. Doubly involute. | Hemispheres larger, convoluted. | Lower (White River.) | 3-3 4-3 4-4 | Digitigrade. Plantigrade. | Grooved. | Interlocking. | Smooth. Faceted. | 4-tubercles, and crested. | ? Singly involute. | Hemispheres small, and larger. | Eocene. Upper (bridger.) | 3-3 4-3 4-5 5-5 | (Digitigrade.) Plantigrade. | Grooved. (Flat.) | Opposite. Interlocking. | Smooth. | 4-tubercles. 3-tubercles, and crested. | Singly involute. Plane. | Hemispheres small | Middle. (Wasatch.) | 4-3 4-5 5-5 | Plantigrade. (Digitigrade.) | Flat. (Grooved.) | Opposite. Interlocking. | Smooth. | 4-tubercles. 3-tubercles, a few crested. | Plane. Singly involute. | Hemispheres small; mesencehpalon sometimes exposed. | Lower (Puerco.) | 5-5 | Plantigrade. | Flat. | Opposite. | Smooth. | 3-tubercles. (4-tubercles), none crested. | Plane. | Mesencephalon exposed; hemisphere small and smoother. | The evidence, then, which is furnished by all parts of the vertebral skeleton—whether we have regard to Fishes, Reptiles, Birds, or Mammals—is cumulative and consistent. Nowhere do we meet with any deviation or ambiguity, while everywhere we encounter similar proofs of continuous transformation—proofs which vary only with the varying amount of material which happens to be at our disposal, being most numerous and detailed in those cases where the greatest number of fossil forms has been preserved by the geological record. Here, therefore, we may leave the vertebral skeleton; and, having presented a sample of the evidence as yielded by horns and bones, I will conclude by glancing with similar brevity at the case of shells—which, as before remarked, constitute the only other sufficiently hard or permanent material to yield unbroken evidence touching the fossil ancestry of animals. Of course it will be understood that I am everywhere giving merely samples of the now superabundant evidence which is yielded by palÆontology; and, as this chapter is already a long one, I must content myself with citing only the case of mollusk-shells, although shells of other classes might be made to yield highly important additions to the testimony. Moreover, even as regards the one division of mollusk-shells, I can afford to quote only a very few cases. These, however, are in my opinion the strongest single pieces of evidence in favour of transmutation which have thus far been brought to light. Near the village of Steinheim, in WÜrtemberg, there is an ancient lake-basin, dating from Tertiary times. The lake has long ago dried up; but its aqueous deposits are extraordinarily rich in fossil shells, especially of different species of the genus Planorbis. The following is an authoritative rÉsumÉ of the facts. As the deposits seem to have been continuous for ages, and the fossil shells very abundant, this seemed to be an excellent opportunity to test the theory of derivation. With this end in view, they have been made the subject of exhaustive study by Hilgendorf in 1866, and by Hyatt in 1880. In passing from the lowest to the highest strata the species change greatly and many times, the extreme forms being so different that, were it not for the intermediate forms, they would be called not only different species, but different genera. And yet the gradations are so insensible that the whole series is nothing less than a demonstration, in this case at least, of origin of species by derivation with modifications. The accompanying plate of successive forms (Fig. 89), which we take from Prof. Hyatt’s admirable memoir, will show this better than any mere verbal explanation. It will be observed that, commencing with four slight varieties—probably sexually isolated varieties—of one species, each series shows a gradual transformation as we go upward in the strata—i. e. onward in time. Series I branches into three sub-series, in two of which the change of form is extreme. Series IV is remarkable for great increase in size as well as change in form. In the plate we give only selected stages, but in the fuller plates of the memoir, and still more in the shells themselves, the subtilest gradations are found[19]. Here is another and more recently observed case of transmutation in the case of mollusks. The recent species, Strombus accipitrinus, still inhabits the coasts of Florida. Its extinct prototype, S. Leidy, was discovered a few years ago by Prof. Heilprin in the Pliocene formations of the interior of Florida. The peculiar shape of the wing, and tuberculation of the whorl, are thus proved to have grown but of a previously more conical form of shell. Transformation of Strombus. Fig. 90.—Transformation of Strombus. (After Heilprin.) 1, 1a, Strombus Leidy (1, typical), Pliocene; 2, 2a, Strombus accipitrinus (2a typical) Recent.
Lastly, attention may here again be directed to the very instructive series of shells which has already been shown in a previous chapter, and which serves to illustrate the successive geological forms of Paludina from the Tertiary beds of Slavonia, as depicted by Prof. Neumayr of Vienna. (Fig. 1, p. 19.)
|
  |