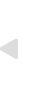
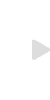
Among the most venerable of natural dye-stuffs is indigo, the substance from which Unverdorben first obtained aniline in 1826. The colouring matter is found in a number of leguminous (see Fig. 7), cruciferous, and other plants, some of which are largely cultivated in India, China, the Malay Archipelago, South America, and the West Indies; while others, such as woad (see Fig. 8), are grown in more temperate European climates. The tinctorial value of these plants was known in India and Egypt long before the Christian era. Egyptian mummy-cloths have been found dyed with indigo. The dye was known to the Greeks and Romans; its use is described by the younger Pliny in his Natural History. Indigo was introduced into Europe about the sixteenth century, but its use was strongly opposed by the woad cultivators, with whose industry the dye came into competition. In France the opposition was strong enough to secure the passing of an act in the time of Henry IV. inflicting the penalty of death upon any person found using the dye. The importance of indigo as an article of commerce is sufficiently known at the present time; more than 8000 tons are produced annually, corresponding in money value to about four million pounds. It is of importance to us as rulers of India to remember that the cultivation and manufacture of indigo is one of the staple industries of that country, from which the European markets derive the greater part of their supply.
Fig. 7.—Indigo Plant
(Indigofera tinctoria).
Imagine the industrial revolution which would be caused by the discovery of a process for obtaining indigo synthetically from a coal-tar hydrocarbon, at a price which would compare favourably with that of the natural product. This has not actually been done as yet, but chemists have attempted to compete with Nature in this direction, and the present state of the competition is that the natural product can be cultivated and made more cheaply. Nevertheless the dye can be synthesised from a coal-tar hydrocarbon, and this is one of the greatest achievements of modern chemistry in connection with the tar-products. For more than half a century indigo had been undergoing investigation by chemists, and at length the work culminated in the discovery of a method for producing it artificially. This discovery was the outcome of the labour of Adolf v. Baeyer, who commenced his researches upon the derivatives of indigo in 1866, and who in 1880 secured the first patents for the manufacture of the colouring-matter. It is to the laborious and brilliant investigations of this chemist that we owe nearly all that is at present known about the chemistry of indigo and allied compounds.
Fig. 8.—Woad
(Isatis tinctoria).
Two methods have been used for the production of artificial indigo—benzal chloride being the starting-point in one of these, and nitrobenzoic aldehyde in the other. The generating hydrocarbon is therefore toluene. By heating benzal chloride with dry sodium acetate there is formed an acid known as cinnamic acid, a fragrant compound which derives its name from cinnamon, because the acid was prepared by the oxidation of oil of cinnamon by Dumas and Peligot in 1834. The acid and its ethers occur also in many balsams, so that we have here another instance of the synthesis of a natural vegetable product from a coal-tar hydrocarbon. The subsequent steps are—(1) the nitration of the acid to produce nitrocinnamic acid; (2) the addition of bromine to form a dibromide of the nitro-acid; (3) the action of alkali on the dibromide to produce what is known as “propiolic acid.” The latter, under the influence of mild alkaline reducing agents, is transformed into indigo-blue. The process depending on the use of nitrobenzoic aldehyde is much simpler; but the particular nitro-derivative of the aldehyde which is required is at present difficult to make, and therefore expensive. If the production of this compound could be cheapened, the competition between artificial and natural indigo would assume a much more serious aspect.[5]
The light oil of the tar-distiller has now been sufficiently dealt with so far as regards colouring-matters; let us pass on to the next fraction of the tar, the carbolic oil. The important constituents of this portion are carbolic acid and naphthalene. The carbolic oil is in the first place separated into two distinct portions by washing with an alkaline solution. Carbolic acid or phenol belongs to a class of compounds derived from hydrocarbons of the benzene and related series by the substitution of the residue of water for hydrogen. This water-residue is known to chemists as “hydroxyl”—it is water less one atom of hydrogen. Carbolic acid or phenol is hydroxybenzene; and all analogous compounds are spoken of as “phenols.” It will be understood in future that a phenol is a hydroxy-derivative of a benzenoid hydrocarbon. Now these phenols are all more or less acid in character by virtue of the hydroxyl-group which they contain. For this reason they dissolve in aqueous alkaline solutions, and are precipitated therefrom by acids. This will enable us to understand the purification of the carbolic oil.
The two layers into which this oil separates after washing with alkali are (1) the aqueous alkaline solution of the carbolic acid and other phenols, and (2) the undissolved naphthalene contaminated with oily hydrocarbons and other impurities. Each of these portions has its industrial history. The alkaline solution, on being drawn off and made acid, yields its mixture of phenols in the form of a dark oil from which carbolic acid is separated by a laborious series of fractional distillations. The undissolved hydrocarbon is similarly purified by fractional distillation, and furnishes the solid crystalline naphthalene. The tar from one ton of Lancashire coal yields about 1½ lbs. of carbolic acid, equal to about 1 per cent. by weight of the tar, and about 6¼lbs. of naphthalene, so that this last hydrocarbon is one of the chief constituents of the tar, of which it forms from 8 to 10 per cent. by weight.
The crude carbolic acid as separated from the alkaline solution is a mixture of several phenolic compounds, and all of these but the carbolic acid itself are gradually removed during the process of purification. Among the compounds associated with the carbolic acid are certain phenols of higher boiling-point, which bear the same relationship to carbolic acid that toluene bears to benzene. That is to say, that while phenol itself is hydroxybenzene, these other compounds, which are called “cresols,” are hydroxytoluenes. The cresols form an oily liquid largely used for disinfecting purposes under the designation of “liquid carbolic acid,” or “cresylic acid.” Carbolic acid is a white crystalline solid possessing strongly antiseptic properties, and is therefore of immense value in all cases where putrefaction or decay has to be arrested. It was discovered in coal-tar by Runge in 1834, and was obtained pure by Laurent in 1840.
The gradual establishment of the germ-theory of disease, chiefly due to the labours of Pasteur, has led to a most important application of carbolic acid. Once again we find the coal-tar industry brought into contact with another department of science. Arguing from the view that putrefactive change is brought about by the presence of the germs of micro-organisms ever present in the atmosphere, Sir Joseph Lister proposed that during surgical operations the incised part should be kept under a spray of the germicidal carbolic acid to prevent subsequent mortification. No operation upon portions of the body exposed to the air is at present conducted without this precaution, and many a human life must have been saved by Lister’s treatment. To this result the chemist and technologist have contributed, not only by the discovery of the carbolic acid in the tar, but also by the development of the necessary processes for its purification. It should be added that the phenol used must be of the greatest possible purity, and the requirements of the surgeon have been met by chemical and technological skill.
From surgery back to colouring-matters, and from these to pharmaceutical preparations and perfumes, are we led in following up the cycles of chemical transformation which these tar-products have undergone in the hands of the technologist, guided by the researches of the chemist. It was observed by Runge in 1834 that crude carbolic acid, on treatment with lime, gave a red, acid colouring-matter which he separated and named “rosolic acid.” The observation was followed up, and many other chemists obtained red colouring-matters by the oxidation of crude phenol. In 1859, the colour-giving property of carbolic acid acquired industrial importance from a discovery made by Kolbe and Schmitt in Germany, and by Persoz in France. These chemists found that a good yield of the colouring-matter was obtained by heating phenol with oxalic and sulphuric acids. Under the names of “corallin” and “aurin” the dye-stuff was introduced into commerce, and it is still used for certain purposes, especially for the preparation of coloured lakes for paper-staining.The scientific development of the history of this phenol dye is full of interest, but we can only give it a passing glance. Its interest lies chiefly in the circumstance that it is related to magenta, as was first pointed out by Caro and Wanklyn in 1866. In fact they obtained rosolic acid from magenta by the action of nitrous acid on the latter. We now know that a diazo-salt is first formed under these circumstances, and that the decomposition of this unstable compound in the presence of water gives rise to the rosolic acid. Later researches have shown that by heating rosolic acid with ammonia it is converted into rosaniline. It is also known that the commercial corallin, like the commercial magenta, is a mixture of closely related colouring-matters. The close analogy between magenta and rosolic acid was further shown by Caro in 1866. In the same way that Hofmann found that magenta could not be produced by the oxidation of pure aniline, Caro found that a mixture of phenol and cresol was necessary for the production of rosolic acid when inorganic oxidizers were used. It is indeed this series of investigations upon the phenol dyes—investigations which have been taken part in not only by the chemists named, but also by Graebe, Dale and Schorlemmer, and the Fischers—which led up to the discovery of the constitution of the colouring-matters of the rosaniline group, and, through this, to the far-reaching industrial developments of the discovery as traced in the last chapter. It is evident, from what has been said, that rosolic acid and its related colouring-matters are members of the triphenylmethane group. They are in fact the hydroxylic or acid analogues of the amido-containing or basic dyes of the rosaniline series.
In the fragrant blossom of the meadowsweet (SpirÆa ulmaria) there is contained an acid which is found also as an ether in the oil of wintergreen (Gautheria procumbens). This is salicylic acid, a white crystalline compound which has been known to chemists since 1839. In 1860 Kolbe prepared the sodium salt of this acid by passing carbon dioxide gas into phenol in which metallic sodium had been dissolved. It was found subsequently that the same transformation was brought about by heating the dry sodium salt of carbolic acid in an atmosphere of carbon dioxide. This process of Kolbe’s is now worked on a manufacturing scale for the preparation of artificial salicylic acid. The acid and its salts and ethers find numerous applications as antiseptics, for the preservation of food, and in pharmacy.
Salicylic acid is employed also for the manufacture of certain azo-dyes in a way that it will be very instructive to consider, because the process used may be taken as typical of the general method of preparing such compounds. Solutions of diazo-salts act not only upon amido- and diamido-compounds, as we have seen in the case of aniline yellow and chrysoÏdine, but also upon phenols, forming acid azo-colours. This important fact was made known in 1870 by the German chemists KekulÉ and Hidegh, but more than six years elapsed before this discovery was taken advantage of by the technologist. Large numbers of these acid azo-dyes are now made from various diazotised amido-compounds combined with different phenols and phenolic acids. The mode of procedure is to diazotise the amido-compound by sodium nitrite and hydrochloric acid in the manner already described, and then add the diazo-salt solution to the phenolic compound dissolved in alkali. The colouring-matter is at once formed. Salicylic acid possesses the characters both of an acid and a phenol. It combines readily with diazo-salts under the circumstances described, and gives rise to azo-dyes, some of which are of technical value.
The manufacture of azo-dyes from salicylic acid brings us into contact with certain amidic compounds which figure so largely in the tinctorial industry that they may be conveniently dealt with here. These bases are not azo-compounds themselves, but they are prepared from azo-compounds, viz. from the azobenzene and azotoluene which were spoken about in the last chapter. When these are reduced by acid reducing-agents, they become converted into diamido-bases which are known as benzidine and tolidine respectively. These bases can be diazotised, and as they contain two amido-groups, they form double diazo-salts, i.e. tetrazo-salts, which are capable of combining with amido-compounds, or phenols, in the usual way. Thus diazotised benzidine and tolidine combine with salicylic acid to form valuable yellow azo-dyes known as “chrysamines.” The dyes of this class obviously contain two azo-groups.
Some other uses of carbolic acid must next be considered. Of the colouring-matters derived from coal-tar, none is more widely known than the oldest artificial yellow dye, picric acid. This is a phenol derivative, and was first obtained as long ago as 1771 by Woulfe, by acting upon indigo with nitric acid. Laurent in 1842 was the first to obtain this dye from carbolic acid, from which compound it is still manufactured by acting upon the sulpho-acid with nitric acid. Chemically considered, it is trinitrophenol. It has a very wide application as a dye, and has been used as an explosive agent. A similar colouring-matter was made from cresol in 1869, and introduced under the name of “Victoria yellow,” which is dinitro-cresol. Other dyes derived directly or indirectly from phenol will take us back once again to toluene.
A new diazotisable diamido-compound was obtained from this last hydrocarbon, and introduced in 1886 by Leonhardt & Co. One of the three isomeric nitrotoluenes furnishes a sulpho-acid which, on treatment with alkali, gives a compound derived from a hydrocarbon known as stilbene, and this, on reduction, is converted into the diamido-compound referred to. The latter, which is a disulpho-acid as well as a diamido-compound, can be diazotised and combined with phenols, &c. The stilbene azo-dyes thus prepared from phenol and salicylic acid, like the chrysamines, are yellow colouring-matters, containing two azo-groups. It is a valuable characteristic of these secondary azo-dyes that they all possess a special affinity for vegetable fibre, and their introduction has exerted a great influence upon the art of cotton-dyeing. We shall have to return to these cotton-dyes again shortly.
Before leaving this branch of the subject, the following scheme is presented to show the relationships and inter-relationships of the products thus far dealt with in the present chapter—
The existence of naphthalene in coal-tar was made known in 1820 by Garden, who gave it this name because the oils obtained from the tar by distillation went under the general designation of naphtha. The greater portion of the hydrocarbon is contained in the carbolic oil, and is separated and purified in the manner described. A further quantity of impure naphthalene separates out from the next fraction—the creosote oil, and this is similarly washed and purified by distillation. The large quantity of naphthalene existing in tar has already been referred to, but although it is such an important constituent, it was only late in the history of the colour industry that it found any extensive application. In early times it was regarded as a nuisance, and was burnt as fuel, or for the production of a dense soot, which was condensed to form lampblack. It will be remembered that the first of the coal-tar colours made required only the light oils. There are at present only a few direct uses for naphthalene, but one of its applications is sufficiently important to be mentioned.
The hydrocarbon is a white crystalline solid melting at 80° C., and boiling at 217° C. Although it has a high boiling-point, it passes readily into vapour at lower temperatures, and the vapour on condensation forms beautiful silvery crystalline scales. This product is “sublimed naphthalene.” The vapour of naphthalene burns with a highly luminous flame, and if mixed with coal-gas, it considerably increases the luminosity of the flame. Advantage is taken-of this in the so-called “albo-carbon light,” which is the flame of burning coal-gas saturated with naphthalene vapour. The burner is constructed so that the gas passes through a reservoir filled with melted naphthalene kept hot by the flame itself (Fig. 9).
Fig. 9.—Albo-carbon Burner.
To appreciate properly the value of those discoveries which have enabled manufacturers to utilize this hydrocarbon, it is only necessary to recall to mind the actual quantity produced in this country. Supposing that ten million tons of coal are used annually for gas-making, and that the 500,000 tons of tar resulting therefrom contain only eight per cent. of naphthalene, there would be available about 40,000 tons of this hydrocarbon annually. Great as have been the recent advancements in the utilization of naphthalene derivatives, there is still a larger quantity of this hydrocarbon produced than is necessary to supply the wants of the colour-manufacturer. From this last statement it will be inferred that naphthalene is now a source of colouring-matters. Let us consider how this has been brought about.
The phenols of naphthalene are called naphthols—they bear the same relationship to naphthalene that carbolic acid bears to benzene. Owing to the structure of the naphthalene molecule there are two isomeric naphthols, whereas there is only one phenol. The naphthols—known as alpha- and beta-naphthol, respectively—are now made on a large scale from naphthalene, by heating the latter with sulphuric acid; at a low temperature the alpha sulpho-acid is produced, and at a higher temperature the beta sulpho-acid, and these acids on fusion with caustic soda furnish the corresponding naphthols. Similarly there are two amidonaphthalenes, known as alpha- and beta-naphthylamine respectively. As aniline is to benzene, so are the naphthylamines to naphthalene. The alpha-compound is made in precisely the same way as aniline, viz. by acting upon naphthalene with nitric acid so as to form nitronaphthalene, and then reducing the latter with iron dust and acid. Beta-napthylamine cannot be made in this way; it is prepared from beta-naphthol by heating the latter in presence of ammonia, when the hydroxyl becomes replaced by the amido-group in accordance with a process patented in 1880 by the Baden Aniline Company. The principle thus utilized is the outcome of the scientific work of two Austrian chemists, Merz and Weith. Setting out from the naphthols and naphthylamines we shall be led into industrial developments of the greatest importance.
The first naphthalene colour was a yellow dye, discovered by Martius in 1864, and manufactured under the name of “Manchester yellow.” It is, chemically speaking, dinitro-alpha-naphthol; but it was not at first made from naphthol, as the latter was not at the time a technical product. It was made from alpha-naphthylamine by the action of nitrous and nitric acids. When a good method for making the naphthol was discovered in 1869, the dye was made from this. The process is just the same as that employed in making picric acid; the naphthol is converted into a sulpho-acid, and this when acted upon by nitric acid, gives the colouring-matter. Manchester yellow is now largely used for colouring soap, but as a dye-stuff it has been improved upon in a manner that will be readily understood. The original colouring-matter being somewhat fugitive, it was found that its sulpho-acid was much faster. This sulpho-acid cannot be made by the direct action of sulphuric acid upon the colouring-matter—as in the case of acid yellow or acid magenta—but by acting upon the naphthol with very strong sulphuric acid, three sulphuric acid residues or sulpho-groups enter the molecule, and then on nitration only two of these are replaced by nitro-groups, and there results a sulpho-acid of dinitro-alpha-naphthol. This was discovered in 1879 by Caro, and introduced as “acid naphthol yellow.” It is now one of the standard yellow dyes.
The history of another important group of colouring-matters dependent on naphthalene begins with A. v. Baeyer in 1871 and with Caro in 1874. Two products formerly known only as laboratory preparations were called into requisition by this discovery. One of these compounds, phthalic acid, is obtained from naphthalene, and the other, resorcin or resorcinol, is prepared from benzene. Phthalic acid, which was discovered in 1836 by Laurent, is a product of the oxidation of many benzenoid compounds. Chemically considered it is a di-derivative of benzene, i.e. two of the hydrogen atoms of benzene are replaced by certain groups of carbon, oxygen, and hydrogen atoms. We have seen how the replacement of hydrogen by an ammonia-residue, amidogen, gives rise to bases such as amidobenzene (aniline), or diamidobenzene. Similarly, the replacement of hydrogen by a water-residue, hydroxyl, gives rise to a phenol. The group of carbon, oxygen, and hydrogen atoms which confers the property of acidity upon an organic compound is a half-molecule of oxalic acid—it is known as the carboxyl group. Thus benzoic acid is the carboxyl-derivative of benzene, and the phthalic acid with which we are now concerned is a dicarboxyl-derivative of benzene. It is related to benzoic acid in the same way that diamidobenzene is related to aniline. Three isomeric phthalic acids are known, but only one of these is of use in the present branch of manufacture. The acid in question, although a derivative of benzene, is most economically prepared by the oxidation of certain derivatives of naphthalene which, when completely broken down by energetic oxidizing agents, furnish the acid. Thus the dinitronaphthol described as Manchester yellow, if heated for some time with dilute nitric acid, furnishes phthalic acid. The latter is made on a large scale by the oxidation of a compound which naphthalene forms with chlorine, and known as naphthalene tetrachloride, because it contains four atoms of chlorine.
The other compound, resorcinol, was known to chemistry ten years before it was utilized as a source of colouring-matters. It was originally prepared by fusing certain resins, such as galbanum, asafoetida, &c., with caustic alkali. Soon after its discovery, viz. in 1866, it was shown by KÖrner to be a derivative of benzene, and from this hint the technical process for the preparation of the compound on a large scale has been developed. Resorcinol is a phenolic derivative of benzene containing two hydroxyl groups; it is therefore related to phenol in the same way that diamidobenzene is related to aniline or phthalic acid to benzoic acid. The relationships can be expressed in a tabular form thus—
Amidobenzene or Aniline. | | Benzoic acid. | | Carbolic acid or Phenol. |
Diamidobenzene. | | Phthalic acid. | | Resorcinol. |
Resorcinol is now made by heating benzene with very strong sulphuric acid so as to convert it into a disulpho-acid, and the sodium salt of the latter is then fused with alkali. As a technical operation it is one of great delicacy and skill, and the manufacture is confined to a few Continental factories.When phthalic acid is heated it loses water, and is transformed into a white, magnificently crystalline substance known as phthalic anhydride, i.e. the acid deprived of water. In 1871, A. v. Baeyer, the eminent chemist who subsequently synthesised indigo, published the first of a series of investigations describing the compounds produced by heating phthalic anhydride with phenols. To these compounds he gave the name of “phthaleÏns.” Baeyer’s work, like that of so many other chemists who have contributed to the advancement of the coal-tar colour industry, was of a purely scientific character at first, but it soon led to technological developments. The phthaleÏns are all acid compounds possessing more or less tinctorial power. One of the first discovered was produced by heating phthalic anhydride with an acid known as gallic acid, which occurs in vegetable galls, and in the form of tannin in many vegetable extracts which are used by the tanner. The acid is a phenolic derivative of benzoic acid, viz. trihydroxybenzoic acid, and on heating it readily passes into trihydroxybenzene, which is the “pyrogallic acid” or pyrogallol familiar as a photographic developer. The phthaleÏn formed from gallic acid and phthalic anhydride really results from the union of the latter with pyrogallol. It is now manufactured under the name of “galleÏn,” and is largely used for imparting a bluish grey shade to cotton fabrics. By heating galleÏn with strong sulphuric acid, it is transformed into another colouring-matter which gives remarkably fast olive-green shades when dyed on cotton fibre with a suitable mordant. This derivative of galleÏn is used to a considerable extent under the name of “coeruleÏn.” These two colouring-matters were the first practical outcome of v. Baeyer’s researches. There is another possible development in this direction which chemistry may yet accomplish, and another natural colouring-matter may be threatened, even as the indigo culture was threatened by the later work of the same chemist. There is reason for believing that the colouring-matter of logwood, known to chemists as hÆmateÏn, is related to or derived in some way from the phthaleÏns, and the synthesis of this compound may ultimately be effected.
The dye introduced by Caro in 1874 is the brominated phthaleÏn of resorcinol. The phthaleÏn itself is a yellow dye, and the solutions of its salts show a splendid and most intense greenish yellow fluorescence, for which reason it is called “fluoresceÏn.” When brominated, the latter furnishes a beautiful red colouring-matter known as “eosin” (Gr. ???, dawn), and the introduction of this gave an industrial impetus to the phthaleÏns which led to the discovery of many other related colouring-matters now largely used under various trade designations. About a dozen distinct compounds producing different shades of pink, crimson and red, and all derived from fluoresceÏn, are at present in the market, and a few other phthaleÏns formed by heating phthalic anhydride with other phenolic compounds instead of resorcinol or pyrogallol (e.g. diethylamidophenol), are also of industrial importance. By converting nitrobenzene into a sulpho-acid, reducing to an amido-sulpho-acid, and then fusing with alkali, an amido-phenol is produced, the ethers of which, when heated with phthalic anhydride, give rise to red phthaleÏns of most intense colouring power introduced by the Baden Aniline Company as “rhodamines.”
It remains to point out that the scientific spirit which prompted the investigation of the phthaleÏns in the first instance has followed these compounds throughout their technological career. The researches started by v. Baeyer were taken up by various chemists, whose work together with that of the original discoverer has led to the elucidation of the constitution of these colouring-matters. The phthaleÏns are members of the triphenylmethane group, and are therefore related to magenta, corallin, malachite green, methyl violet, and the phosgene dyes.
It has been said that the phenolic and amidic derivatives of naphthalene, i.e. the naphthols and naphthylamines, are of the greatest importance to the colour industry. One of the first uses of alpha-naphthylamine has already been mentioned, viz. for the production of the Manchester yellow, which was afterwards made more advantageously from alpha-naphthol. A red colouring-matter possessing a beautiful fluorescence was afterwards (1869) made from this naphthylamine and introduced as “Magdala red.” The latter was discovered by Schiendl of Vienna in 1867. It was prepared in precisely the same way as induline was prepared from aniline yellow. The latter, which is amido-azobenzene, and which is prepared, broadly speaking, by the action of nitrous acid on aniline, has its analogue in amido-azonaphthalene, which is similarly prepared by the action of nitrous acid on naphthylamine. Just as aniline yellow when heated with aniline and an aniline salt gives induline, so amido-azonaphthalene when heated with naphthylamine and a salt of this base gives Magdala red. The latter is, therefore, a naphthalene analogue of induline, as was shown by Hofmann in 1869, and the knowledge of the constitution of the azines which has been gained of late years, enables us to relegate the colouring-matter to this group. This knowledge has also enabled the manufacture to be conducted on more rational principles, viz. by the method employed for the production of the saffranines, as previously sketched.
The introduction of azo-dyes, formed by the action of a diazotised amido-compound on a phenol or another amido-compound, marks the period from which the naphthols and naphthylamines rose to the first rank of importance as raw materials for the colour manufacturer. The introduction of chrysoÏdine in 1876 was immediately followed by the manufacture of acid azo-dyes obtained by combining diazotised amido-sulpho-acids with phenols of various kinds, or with bases, such as dimethylaniline and diphenylamine. From what has been said in the foregoing portion of this volume, it is evident that all such azo-compounds result from the combination of two things, viz. (1) a diazotised amido-compound, and (2) a phenolic or amidic compound. Either (1) or (2) or both may be a sulpho-acid, and the resulting dye will then also be a sulpho-acid.
The first of these colouring-matters derived from the naphthols, was introduced in 1876-77 by Roussin and Poirrier, and by O. N. Witt. They were prepared by converting aniline into a sulpho-acid (sulphanilic acid), diazotising this and combining the diazo-compound with alpha- or beta-naphthol. The compounds formed are brilliant orange dyes, the latter being still largely consumed as “naphthol orange.” Other dye-stuffs of a similar nature were introduced by Caro about the same time, and were prepared from the diazotised sulpho-acid of alpha-naphthylamine combined with the naphthols. By this means alpha-naphthol gives what is known as “acid brown,” or “fast brown,” and beta-naphthol a fine crimson, known as “fast red,” or “roccellin.” Diazotised compounds combine also with this same sulpho-acid of alpha-naphthylamine (known as naphthionic acid), the first colouring-matter formed in this way having been introduced by Roussin and Poirrier in 1878. It was prepared by diazotising a nitro-derivative of aniline, and acting with the diazo-salt on napthionic acid, and this dye is still used to some extent under the name of “archil substitute.” In 1878, the firm of Meister, Lucius & BrÜning of HÖchst-on-the-Main gave a further impetus to the utilization of naphthalene by discovering two isomeric disulpho-acids of beta-naphthol formed by heating that phenol with sulphuric acid. By combining various diazotised bases with these sulpho-acids, a splendid series of acid azo-dyes ranging in shade from bright orange to claret-red, and to scarlets rivalling cochineal in brilliancy were given to the tinctorial industry.
The colouring-matters introduced in 1878 by the HÖchst factory under the names of “Ponceaux” of various brands, and “Bordeaux,” although to some extent superseded by later discoveries, still occupy an important position. Their discovery not only increased the consumption of beta-naphthol, but also that of the bases which were used for diazotising. These bases are alpha-naphthylamine and those of the aniline series. The intimate relationship which exists between chemical science and technology—a relationship which appears so constantly in the foregoing portions of this work—is well brought out by the discovery under consideration. A little more chemistry will enable this statement to be appreciated.
Going back for a moment to the hydrocarbons obtained from light oil, it will be remembered that benzene and toluene have thus far been considered as the only ones of importance to the colour-maker. Until the discovery embodied in the patent specification of 1878, the portions of the light oil boiling above toluene were of no value in the colour industry. Benzene and toluene are related to each other in a way which chemists describe by saying that they are “homologous.” This means that they are members of a regularly graduated series, the successive terms of which differ by the same number of atoms of carbon and hydrogen. Thus toluene contains one atom of carbon and two atoms of hydrogen more than benzene. Above toluene are higher homologues, viz. xylene, cumene, &c., which occur in the light oil, the former being related to toluene in the same way that toluene is related to benzene, while cumene again contains one atom of carbon and two atoms of hydrogen more than xylene. This relationship between the members of homologous series is expressed in other terms by saying that the weight of the molecule increases by a constant quantity as we ascend the series.
The homology existing among the hydrocarbons extends to all their derivatives. Thus phenol is the lower homologue of the cresols. Also we have the homologous series—
Benzene. | | Nitrobenzene. | | Aniline. |
Toluene. | | Nitrotoluene. | | Toluidine. |
Xylene. | | Nitroxylene. | | Xylidine. |
Cumene. | | Nitrocumene. | | Cumidine. |
The bases of the third column when diazotised and combined with the disulpho-acids of beta-naphthol give a graduated series of dyes beginning with orange and ending with bluish scarlet. Thus it was observed that the toluidine colour was redder than the aniline colour, and it was a natural inference that the xylidine colour would be still redder. At the time of this discovery no azo-colour of a true scarlet shade had been manufactured successfully. A demand for the higher homologues of benzene was thus created, and the higher boiling-point fractions of the light oil, which had been formerly used as solvent naphtha, became of value as sources of colouring-matters. The isolation of coal-tar xylene (which is a mixture of three isomeric hydrocarbons) is easily effected by fractional distillation with a rectifying column, and by nitration and reduction, in the same way as in the manufacture of aniline, xylidine is placed at the disposal of the colour-maker.
Xylidine scarlet, although at the time of its introduction the only true azo-scarlet likely to come into competition with cochineal, was still somewhat on the orange side. The cumidine dye would obviously be nearer the desired shade. To meet this want, cumidine had to be made on a large scale, but here practical difficulties interposed themselves. The quantity of cumene in the light oil is but small, and it is associated with other hydrocarbons which are impurities from the present point of view, and from which it is separated only with difficulty. A new source of the base had therefore to be sought, and here again we find chemical science ministering to the wants of the technologist.
It was explained in the last chapter that aniline and similar bases can be methylated by heating their dry salts with methyl alcohol under pressure. In this way dimethylaniline is made, and dimethyltoluidine or dimethylxylidine can similarly be prepared. Now it was shown by Hofmann in 1871, that if this operation is conducted at a very high temperature, and under very great pressure, the methyl-alcohol residue, i.e. the methyl-group, does not replace the amidic hydrogen or the hydrogen of the ammonia residue, but the methylation takes place in another way, resulting in the formation of a higher homologue of the base started with. For example, by heating aniline salt and pure wood-spirit to a temperature considerably above that necessary for producing dimethylaniline, toluidine is formed. In a similar way, by heating xylidine hydrochloride and methyl alcohol for some time in a closed vessel at about 300° C. cumidine is produced. Hofmann’s discovery was thus utilized in 1882, and by its means the base was manufactured, and cumidine scarlet, very similar in shade to cochineal, became an article of commerce.
While the development of this branch of the colour industry was taking place by means of the new naphthol disulpho-acids, the cultivation of the fertile field of the azo-dyes was being carried on in other directions. It came to be realized that the fundamental discovery of Griess was capable of being extended to all kinds of amido-compounds. The azo-dyes hitherto introduced had all been derived from amido-compounds containing only one amido-group, and they accordingly contained only one azo-group; they were primary azo-compounds. It was soon found that aniline yellow, which already contains one azo-group as well as an amido-group, could be again diazotised and combined with phenols so as to produce compounds containing two azo-groups, i.e. secondary azo-compounds. The sulpho-acid of aniline yellow—GrÄssler’s “acid yellow”—was the first source of azo-dyes of this class. By diazotising this amidoazo-sulpho-acid, and combining it with beta-naphthol, a fine scarlet dye was discovered by Nietzki in 1879, and introduced under the name of “Biebrich scarlet.” Two years later a new sulpho-acid of beta-naphthol was discovered by Bayer & Co. of Elberfeld, and this gave rise, when combined with diazotised acid yellow and analogous compounds, to another series of brilliant dyes introduced as “Crocein scarlets.”
From these beginnings the development of the azo-dyes has been steadily carried on to the present time—year by year new diazotisable amido-compounds or new sulpho-acids of the naphthols and naphthylamines are being discovered, and this branch of the colour industry has already assumed colossal dimensions. An important departure was made in 1884 by BÖttiger, who introduced the first secondary azo-colours derived from benzidine. As already explained in connection with salicylic acid, this base and its homologue tolidine form tetrazo-salts, which combine with phenols and amines or their sulpho-acids. One of the first colouring-matters of this group was obtained by combining diazotised benzidine with the sulpho-acid of alpha-naphthylamine (naphthionic acid), and was introduced under the name of “Congo red.” Then came the discovery (Pfaff, 1885), that the tetrazo-salts of benzidine and tolidine combine with phenols, amines, &c., in two stages, one of the diazo-groups first combining with one-half of the whole quantity of phenol to form an intermediate compound, which then combines with the other half of the phenol to form the secondary azo-dye. In the hands of the “Actiengesellschaft fÜr Anilinfabrikation” of Berlin this discovery has been utilized for the production of a number of such azo-colours containing two distinct phenols, or amines, or sulpho-acids. Tolidine has been found to give better colouring-matters in most cases than benzidine, and it is scarcely necessary to point out that an increased demand for the nitrotoluene from which this base is made is the necessary consequence of this discovery.
It is impossible to attempt to specify by name any of these recent benzidine and tolidine dyes. Their introduction has been the means of finding new uses for the naphthylamines and naphthols and their sulpho-acids, and has thus contributed largely to the utilization of naphthalene. An impetus has been given to the investigation of these sulpho-acids, and chemical science has profited largely thereby. The process by which beta-naphthylamine is prepared from beta-naphthol, already referred to, viz. by heating with ammonia under pressure, has been extended to the sulpho-acids of beta-naphthol, and by this means new beta-naphthylamine sulpho-acids have been prepared, and figure largely in the production of these secondary azo-colours. The latter, as previously stated, possess the most valuable property of dyeing cotton fibre directly, and by their means the art of cotton dyeing has been greatly simplified. The shades given by these colours vary from yellow through orange to bright scarlet, violet, or purple.
In addition to benzidine and tolidine, other diazotisable amido-compounds have of late years been pressed into the service of the colour-manufacturer. The derivative of stilbene, already mentioned as being prepared from a sulpho-acid of one of the nitrotoluenes, forms tetrazo-salts, which can be combined with similar or dissimilar phenols, amines, or sulpho-acids, as in the case of benzidine and tolidine. Various shades of red and purple are thus obtained from the diazotised compound, when the latter is combined with the naphthylamines, naphthols, or their sulpho-acids. These, again, are all cotton dyes. The nitro-derivatives of the ethers of phenol and cresol, when reduced in the same way that nitrobenzene and nitrotoluene are reduced to azobenzene and azotoluene, also furnish azo-compounds which, on further reduction, give bases analogous to benzidine and tolidine. Secondary azo-colours derived from these bases and the usual naphthalene derivatives are also manufactured. It is among the secondary azo-dyes that we meet with the first direct dyeing blacks, the importance of which will be realized when it is remembered that the ordinary aniline-black is not adapted for wool dyeing. The azo-blacks are obtained by combining diazotised sulpho-acids of amidoazo-compounds of the benzene or naphthalene series with naphthol sulpho-acids or other naphthalene derivatives.
One other series of azo-compounds must be briefly referred to. It has long been known that aniline and toluidine when heated with sulphur evolve sulphuretted hydrogen and give rise to thio-bases, that is, aniline or toluidine in which the hydrogen is partly replaced by sulphur. One of the toluidines treated in this way is transformed into a thiotoluidine which, when diazotised and combined with one of the disulpho-acids of beta-naphthol, forms a red azo-dye, introduced by Dahl & Co. in 1885 as “thiorubin.” By modifying the conditions of reaction between the sulphur and the base, it was found in 1887 by Arthur Green, that a complicated thio-derivative of toluidine could be produced which possessed very remarkable properties. The sulpho-acid of the thio-base is a yellow dye, which was named by its discoverer “primuline.” Not only is primuline a dye, but it contains an amido-group which can be diazotised. If therefore the fabric dyed with primuline is passed through a nitrite bath, a diazo-salt is formed in the fibre, and on immersing the latter in a second bath containing naphthol or other phenol or an amine, an azo-dye is precipitated in the fibre. By this means there are produced valuable “ingrain colours” of various shades of red, orange, purple, &c.
So much for the azo-dyes, one of the most prolific fields of industrial enterprise connected with coal-tar technology. From the introduction of aniline yellow in 1863 to the present time, about 150 distinct compounds of this group have been given to the tinctorial industry. Of these over thirty are cotton dyes containing two azo-groups. Sombre shades, rivalling logwood black, bright yellows, orange-reds, browns, violets, and brilliant scarlets equalling cochineal, have been evolved from the refuse of the gas-works. The artificial colouring-matters have in this last case once again threatened a natural product, and with greater success than the indigo synthesis, for the introduction of the azo-scarlets has caused a marked decline in the cochineal culture.
In addition to the azo-colours, there are certain other products which claim naphthalene as a raw material. In 1879 it was found that one of the sulpho-acids of beta-naphthol when treated with nitrous acid readily gave a nitroso-sulpho-acid. A salt of this last acid, containing sodium and iron as metallic bases, was introduced in 1884, under the name of “naphthol green.” It is used both as a dye for wool and as a pigment. It may be mentioned here that other nitroso-derivatives of phenols, such as those of resorcinol and the naphthols, under the name of “gambines,” are largely used for dyeing purposes, owing to the facility with which they combine with metallic mordants to form coloured salts in the fibre. In this same year, 1879, it was found that by heating nitrosodimethylaniline with beta-naphthol in an appropriate solvent, a violet colouring-matter was formed. This is now manufactured under the name of “new blue,” or other designations, and is largely used for producing an indigo-blue shade on cotton prepared with a suitable mordant. The discovery of this colouring-matter gave an impetus to further discoveries in the same direction. It was found that nitrosodimethylaniline reacted in a similar way with other phenolic or with amidic compounds. In 1881 KÖchlin introduced an analogous dye-stuff prepared by the action of the same nitroso-compound on gallic acid. Gallocyanin, as it is called, imparts a violet blue shade to mordanted cotton. Other colouring-matters of the same group are in use; some of them, like “new blue,” being derivatives of naphthalene. These compounds all belong to a series of which the parent substance is constructed on a type similar to azine; it contains a nitrogen and oxygen atom linking together the hydrocarbon residues, and is therefore known as “oxazine.” The researches of Nietzki in 1888 first established the true constitution of the oxazines.
Closely related to this group is a colouring-matter introduced by KÖchlin and Witt in 1881 under the name of “indophenol.” It is prepared in the same way as the azines of the “neutral red” group; viz. by the action of nitrosodimethylaniline on alpha-naphthol, or by oxidizing amidodimethylaniline in the presence of alpha-naphthol. Indophenol belongs to that group of blue compounds formed as intermediate products in the manufacture of azines, as mentioned in connection with “neutral red.” But while these intermediate blues resulting from the oxidation of a diamine in the presence of another amine are unstable, and pass readily into red azines, indophenol is stable, and can be used for dyeing and printing in the same way as indigo. The shades which it produces are very similar to this last dye, but for certain practical reasons it has not been able to compete with the natural dye-stuff.
The story of naphthalene is summarized in the schemes on pp. 164, 165.
The fraction of coal-tar succeeding the carbolic oil, viz. the creosote oil, does not at present supply the colour manufacturer with any raw materials beyond the small proportion of naphthalene which separates from it in a very impure condition as “creosote salts.” This oil consists of a mixture of the higher homologues of phenol with various hydrocarbons and basic compounds. It is the oil used for creosoting timber in the manner already described; and among its other applications may be mentioned its use as an illuminating agent and as a source of lampblack. In order to burn the oil effectively as a source of light, a specially-constructed burner is used, which is fed by a stream of oil raised from a reservoir at its foot by means of compressed air, which also aids the combustion of the oil. There is produced by this means a great body of lurid flame, which is very serviceable where building or other operations have to be carried on at night (see Fig. 10). For lampblack the oil is simply burnt in iron pans set in ovens, and the sooty smoke conducted into condensing chambers. The creosote oil constitutes more than 30 per cent. by weight of the tar—the time may come when this fraction, like the light oil and carbolic oil, may be found to contain compounds of value to the colour-maker or to other branches of chemical manufacture.
Fig. 10.—Vertical Burner for heavy Coal Oil
by the Lucigen Light Co.
Fig. 11.—The Madder Plant
(Rubia tinctoria).
The utilization of the next fraction, anthracene oil, is one of the greatest triumphs which applied chemical science can lay claim to since the foundation of the coal-tar colour industry. This discovery dates from 1868, when it was shown by two German chemists, Graebe and Liebermann, that the colouring-matter of madder was derived from the hydrocarbon anthracene. Like indigo, madder may be regarded as one of the most ancient of natural dye-stuffs. It consists of the powdered roots of certain plants of the genus Rubia, such as R. tinctoria (see Fig. 11), R. peregrina, and R. munjista, which were at one time cultivated on an enormous scale in various parts of Europe and Asia. It is estimated that at the time of Graebe and Liebermann’s discovery, 70,000 tons of madder were produced annually in the madder-growing countries of the world. At that time we were importing madder into this country at the rate of 15,000 to 16,000 tons per annum, at a cost of £50 per ton. In ten years the importation had fallen to about 1600 tons, and the price to £18 per ton. At the present time the cultivation of madder is practically extinct.
There is no better gauge of the practical utility of a scientific discovery than the financial effect. In addition to madder, a more concentrated extract containing the colouring-matter itself was largely used by dyers and cotton printers under the name of “garancin.” In 1868 we were importing, in addition to the 15,000 to 16,000 tons of madder, about 2000 tons of this extract annually, at a cost of £150 per ton. By 1878 the importation of garancin had sunk to about 140 tons, and the price had been lowered to £65 per ton. The total value of the imports of madder and garancin in 1868 was over one million pounds sterling; in ten years the value of these same imports had been reduced to about £38,000. Concurrently with this falling off in the demand for the natural colouring-matter, the cultivation of the madder plant had to be abandoned, and the vast tracts of land devoted to this purpose became available for other crops. A change amounting to a revolution was produced in an agricultural industry by a discovery in chemistry.
In the persons of two Frenchmen, Messrs. Robiquet and Colin, science laid hands on the colouring-matter of the Rubia in 1826. These chemists isolated two compounds which they named alizarin and purpurin. It is now known that there are at least six distinct colouring-matters in the madder root, all of these being anthracene derivatives. It is known also that the colouring-matters do not exist in the free state in the plant, but in the form of compounds known as glucosides, i.e. compounds consisting of the colouring-matter combined with the sugar known as glucose. It may be mentioned incidentally that the colouring-matter of the indigo plant also exists as a glucoside in the plant. During a period of more than forty years from the date of its isolation, alizarin was from time to time submitted to examination by chemists, but its composition was not completely established till 1868, when Graebe and Liebermann, by heating it with zinc-dust, obtained anthracene. This was the discovery which gave the death-blow to the madder culture, and converted the last fraction of the tar-oil from a waste product into a material of the greatest value.
The large quantity of madder consumed for tinctorial purposes is indicative of the value of this dye-stuff. It produces shades of red, purple, violet, black, or deep brown, according to the mordant with which the fabric is impregnated. The colours obtained by the use of madder are among the fastest of dyes, the brilliant “Turkey red” being one of the most familiar shades. The discovery of the parent hydrocarbon of this colouring-matter which had been in use for so many ages—a colouring-matter capable of furnishing both in dyeing and printing many distinct shades, all possessed of great fastness—was obviously a step towards the realization of an industrial triumph, viz. the chemical synthesis of alizarin. Within a year of their original observation, this had been accomplished by Graebe and Liebermann, and almost simultaneously by W. H. Perkin in this country. From that time the anthracene, which had previously been burnt or used as lubricating grease, rose in value to an extraordinary extent. In two years a material which could have been bought for a few shillings the ton, rose at the touch of chemical magic to more than two hundred times its former value.
Anthracene is a white crystalline hydrocarbon, having a bluish fluorescence, melting at 213° C. and boiling above 360° C. It was discovered in coal-tar by Dumas and Laurent in 1832, and its composition was determined by Fritzsche in 1857. It separates in the form of crystals from the anthracene oil on cooling, and is removed by filtration. The adhering oil is got rid of by submitting the crystals to great pressure in hydraulic presses. Further purification is effected by powdering the crude anthracene cake and washing with solvent naphtha, i.e. the mixture of the higher homologues of benzene left after the rectification of the light oil. Another coal-tar product, viz. the pyridine base referred to in the last chapter, has been recently employed for washing anthracene with great success. It is used either by itself or mixed with the solvent naphtha. The anthracene by washing with these solvents is freed from more soluble impurities, and may then contain from 30 to 80 per cent. of the pure hydrocarbon. The washing liquid, which is recovered by distillation, contains, among other impurities dissolved out of the crude anthracene, a hydrocarbon isomeric with the latter, and known as phenanthrene, for which there is at present but little use, but which may one day be turned to good account. The actual amount of anthracene contained in coal-tar corresponds to about ½ lb. per ton of coal distilled, i.e. from ¼ to ½ per cent. by weight of the tar. Owing to the great value of alizarin and the large quantity of this colouring-matter annually consumed, anthracene is now by far the most important of the coal-tar hydrocarbons.
Alizarin, purpurin, and the other colouring-matters of madder are hydroxyl derivatives of a compound derived from anthracene by the replacement of two atoms of hydrogen by two atoms of oxygen. These oxygen derivatives of benzenoid hydrocarbons form a special group of compounds known as quinones. Thus there is quinone itself, or benzoquinone, which is benzene with two atoms of oxygen replacing two atoms of hydrogen. There are also isomeric quinones of the naphthalene series known as naphthaquinones. A dihydroxyl derivative of one of the latter is in use under the somewhat misappropriate name of “alizarin black.” With this exception no other quinone derivative is used in the colour industry till we come to the hydrocarbons of the anthracene oil. Phenanthrene forms a quinone which has been utilized as a source of colouring-matters, but these are comparatively unimportant. The quinone with which we are at present concerned is anthraquinone.
The latter is prepared by oxidizing the anthracene—previously reduced by sublimation to the condition of a very finely-divided crystalline powder—with sulphuric acid and potassium dichromate. The quinone is purified, converted into a sulpho-acid, and the sodium salt of the latter on fusion with alkali gives alizarin, which is dihydroxy-anthraquinone. It is of interest to note that in this case a monosulpho-acid gives a dihydroxy-derivative. During the process of fusion potassium chlorate is added, by which means the yield of alizarin is considerably increased. In the original process of Graebe and Liebermann, dibromanthraquinone was fused with alkali; but this method was soon improved upon by the discovery of the sulpho-acid by Caro and Perkin in 1869, and from this period the manufacture of artificial alizarin became commercially successful.
In addition to alizarin, other anthracene derivatives are of industrial importance. The purpurin, discovered among the colouring-matters of madder in 1826, is a trihydroxy-anthraquinone; it can be prepared by the oxidation of alizarin, as shown by De Lalande in 1874. Isomeric compounds known as “flavopurpurin” and “anthrapurpurin” are also made from the disulpho-acids of anthraquinone by fusion with alkali and potassium chlorate. These two disulpho-acids are obtained simultaneously with the monosulpho-acid by the action of fuming sulphuric acid on the quinone, and are separated by the fractional crystallization of their sodium salts from the monosulpho-acid (which gives alizarin) and from each other. The purpurins give somewhat yellower shades than alizarin. Another trihydroxy-anthraquinone, although not obtained directly from anthracene, must be claimed as a tar-product. It is prepared by heating gallic acid with benzoic and sulphuric acids, or with phthalic anhydride and zinc chloride, and is a brown dye known as “anthragallol” or “anthracene-brown.” The anthracene derivative is in this process built up synthetically. A sulpho-acid of alizarin has been introduced for wool dyeing under the name of alizarin carmine, and a nitro-alizarin under the name of alizarin orange. The latter on heating with glycerin and sulphuric acid is transformed into a remarkably fast colouring-matter known as alizarin blue, which is used for dyeing and printing. By heating alizarin blue with strong sulphuric acid, it is converted into alizarin green.
The great industry arising out of the laboratory work of two German chemists has influenced other branches of chemical manufacture, and has reacted upon the coal-tar colour industry itself. A new application for caustic soda and potassium chlorate necessitated an increased production of these materials. The first demand for fuming sulphuric acid on a large scale was created by the alizarin manufacture in 1873, when it was found that an acid of this strength gave better results in the preparation of sulpho-acids from anthraquinone. The introduction of this acid into commerce no doubt exerted a marked influence on the production of other valuable sulpho-acids, such as acid magenta in 1877, acid yellow in 1878, and acid naphthol yellow in 1879. The introduction of artificial alizarin has also simplified the art of colour printing on cotton fabrics to such an extent that other colouring-matters, also derived from coal-tar, are largely used in combination with the alizarin to produce parti-coloured designs. The manufacture of one coal-tar colouring-matter has thus assisted in the consumption of others.
Artificial alizarin is used in the form of a paste, which consists of the colouring-matter precipitated from its alkaline solution by acid, and mixed with water so as to form a mixture containing from 10 to 20 per cent. of alizarin. The magnitude of the industry will be gathered from the estimate that the whole quantity of anthracene annually made into alizarin corresponds to a daily production of about 65 tons of 10 per cent. paste, of which only about one-eighth is made in this country, the remainder being manufactured on the Continent. The total production of alizarin corresponds in money value to about £2,000,000 per annum. One pound of dry alizarin has the tinctorial power of 90 pounds of madder. Seeing therefore that the raw material anthracene was at one time a waste product, and that the quantity of alizarin produced in the factory corresponds to nearly five pounds of 20 per cent. paste for one pound of anthracene, it is not surprising that the artificial has been enabled to compete successfully with the natural product.
The industrial history of anthracene is thus summarized. (See opposite.)
The black, viscid residue left in the tar-still after the removal of the anthracene oil is the substance known familiarly as pitch. From the latter, after removal of all the volatile constituents, there is prepared asphalte, which is a solution of the pitchy residue in the heavy tar-oils from which all the materials used in the colour industry have been removed. Asphalte is used for varnish-making, in the construction of hard pavements, and for other purposes. A considerable quantity of pitch is used in an industry which originated in France in 1832, and which is still carried out on a large scale in that country, and to a smaller extent in this and other tar-producing countries. The industry in question is the manufacture of fuel from coal-dust by moulding the latter in suitable machines with pitch so as to form the cakes known as “briquettes” or “patent fuel.” By this means two waste materials are disposed of in a useful way—the pitch and the finely-divided coal, which could not conveniently be used as fuel by itself. From two to three million tons of this artificial fuel are being made annually here and on the Continent.
The various constituents of coal-tar have now been made to tell their story, so far as relates to the colouring-matters which they furnish. If the descriptive details are devoid of romance to the general reader, the results achieved in the short period of thirty-five years, dating from the discovery of mauve by Perkin, will assuredly be regarded as falling but little short of the marvellous. Although the most striking developments are naturally connected with the colouring-matters, whose history has been sketched in the foregoing pages, and whose introduction has revolutionized the whole art of dyeing, there are other directions in which the coal-tar industry has in recent times been undergoing extension. Certain tar-products are now rendering good service in pharmacy. Salicylic acid and its salts have long been used in medicine. By distilling a mixture of the dry lime salts of benzoic and acetic acids there is obtained a compound known to chemists as acetophenone, which is used for inducing sleep under the name of hypnone. The acetyl-derivative of aniline and of methylaniline are febrifuges known as “antifebrine” and “exalgine.” Ethers of salicylic acid and its homologues, prepared from these acids and phenol, naphthols, &c., are in use as antiseptics under the general designation of “salols.”
In 1881 there was introduced into medicine the first of a group of antipyretics derived from coal-tar bases of the pyridine series. It has already been explained that this base is removed from the light oil by washing with acid. Chemically considered, it is benzene containing one atom of nitrogen in place of a group consisting of an atom of carbon and an atom of hydrogen. The quantity of pyridine present in coal-tar is very small, and no use has as yet been found for it excepting as a solvent for washing anthracene or for rendering the alcohol used for manufacturing purposes undrinkable, as is done in this country by mixing in crude wood-spirit so as to form methylated spirit. The salts of pyridine were shown by McKendrick and Dewar to act as febrifuges in 1881, but they have not hitherto found their way into pharmacy. The chief interest of the base for us centres in the fact that it is the type of a group of bases related to each other in the same way as the coal-tar hydrocarbons. Thus in coal-tar, in addition to pyridine, there is another base known as quinoline, which is related to pyridine in the same way that naphthalene is related to benzene. Similarly there is a coal-tar base known as acridine, which is found associated with the anthracene, and which is related to quinoline in the same way that anthracene is related to naphthalene. The three hydrocarbons are comparable with the three bases, which may be regarded as derived from them in the same manner that pyridine is derived from benzene—
Benzene | | ... | | ... | | ... | | Pyridine |
Naphthalene | | ... | | ... | | Quinoline |
Anthracene | | ... | | ... | | ... | | Acridine |
Some of these bases and their homologues are found in the evil-smelling oil produced by the destructive distillation of bones (Dippel’s oil, or bone oil), and the group is frequently spoken of as the pyridine group. The colouring-matter described as phosphine, obtained as a by-product in the manufacture of magenta (p. 94), is a derivative of acridine, and a yellow colouring-matter discovered by Rudolph in 1881, and obtained by heating the acetyl derivative of aniline with zinc chloride, is a derivative of a homologue of quinoline. This dye-stuff, known as “flavaniline,” is no longer made; but it is interesting as having led to the discovery of the constitution of phosphine by O. Fischer and KÖrner in 1884.The antipyretic medicines which we have first to consider are derivatives of quinoline. This base was discovered in coal-tar by Runge in 1834, and was obtained by Gerhardt in 1842 by distilling cinchonine, one of the cinchona alkaloÏds, with alkali. Now it is of interest to note that the quinoline of coal-tar is of no more use to the technologist than the aniline; these bases are not contained in the tar in sufficient quantity to enable them to be separated and purified with economical advantage. If the colour industry had to depend upon this source of aniline only, its development would have been impossible. But as chemistry enabled the manufacturer to obtain aniline in quantity from benzene, so science has placed quinoline at his disposal. This important discovery was made in 1880 by the Dutch chemist Skraup, who found that by heating aniline with sulphuric acid and glycerin in the presence of nitrobenzene, quinoline is produced. The nitrobenzene acts only as an oxidizing agent; the amido-group of the aniline is converted into a group containing carbon, hydrogen, and nitrogen, i.e. the pyridine group. The discovery of Skraup’s method formed the starting-point of a series of syntheses, which resulted in the formation of many products of technical value. In all these syntheses the fundamental change is the same, viz. the conversion of an amidic into a pyridine group. We may speak of the amido-group as being “pyridised” in such processes. Thus alizarin blue, which is formed by heating nitro-alizarin with glycerin and sulphuric acid, results from the pyridisation of the nitro-group. By an analogous method Doebner and v. Miller prepared a homologue of quinoline (quinaldine) in 1881, by the action of sulphuric acid and a certain modification of aldehyde known as paraldehyde on aniline.
Quinoline and its homologue quinaldine have been utilized as sources of colouring-matters. A green dye-stuff, known as quinoline green, was formerly made by the same method as that employed for producing the phosgene colours by Caro and Kern’s process (p. 106). The phthaleÏn of quinaldine was introduced by E. Jacobsen in 1882 under the name of quinoline yellow, a colouring-matter which forms a soluble sulpho-acid by the action of sulphuric acid.
To return to coal-tar pharmaceutical preparations. At the present time seven distinct derivatives of quinoline, all formed by pyridising the amido-group in aniline, amido-phenols, &c., are known in medicine under such names as kairine, kairoline, thalline, and thermifugine. The mode of preparation of these compounds cannot be entered into here, Kairine, the first of the artificial alkaloÏds, is a derivative of hydroxy-quinoline, which was discovered in 1881 by Otto Fischer. All these quinoline derivatives have the property of lowering the temperature of the body in certain kinds of fevers, and may therefore be considered as the first artificial products coming into competition with the natural alkaloÏd, quinine. There is reason for believing that the latter alkaloÏd, the most valuable of all febrifuges, is related to the quinoline bases, so that if its synthesis is accomplished—as may certainly be anticipated—we shall have to look to coal-tar as a source of the raw materials.
Another valuable artificial alkaloÏd, discovered in 1883 by Ludwig Knorr, claims aniline as a point of departure. When aniline and analogous bases are diazotised, and the diazo-salts reduced in the cold with a very gentle reducing agent, such as stannous chloride, there are formed certain basic compounds, containing one atom of nitrogen and one atom of hydrogen more than the original base. These bases were discovered in 1876 by Emil Fischer, and they are known as hydrazines, the particular compound thus obtained from aniline being phenylhydrazine. By the action of this base on a certain compound ether derived from acetic acid, which is known as aceto-acetic ether, there is formed a product termed “pyrazole,” and this on methylation gives the alkaloÏd in question, which is now well known in pharmacy under the name of “antipyrine.”
While dealing with this first industrial application of a hydrazine, it must be mentioned that the original process by which Fischer prepared these bases was improved upon by Victor Meyer and Lecco in 1883, who discovered the use of a cold solution of stannous chloride for reducing the diazo-chloride to the hydrazine. By this method the manufacture of phenylhydrazine and other hydrazines is effected on a large scale—all kinds of amido-compounds and their sulpho-acids can be diazotised and reduced to their hydrazines. Out of this discovery has arisen the manufacture of a new class of colouring-matters related to the azo-dyes. The hydrazines combine with quinones and analogous compounds with the elimination of water, the oxygen coming from the quinone, and the hydrogen from the hydrazine. The resulting products are coloured compounds very similar in properties to the azo-dyes, and one of these was introduced in 1885 by Ziegler, under the name of “tartrazine.” The latter is obtained by the action of a sulpho-acid of phenylhydrazine on dioxytartaric acid, and is a yellow dye, which is of special interest on account of its extraordinary fastness towards light.
Another direction in which coal-tar products have been utilized is in the formation of certain aromatic compounds which occur in the vegetable kingdom. Thus the artificial production of bitter-almond oil from toluene has already been explained. By heating phenol with caustic alkali and chloroform, the aldehyde of salicylic acid, i.e. salicylic aldehyde, is formed, and this, on heating with dry sodium acetate and acetic anhydride, passes into coumarin, the fragrant crystalline substance which is contained in the Tonka bean and the sweet-scented woodruff. Furthermore, the familiar flavour and scent of the vanilla bean, which is due to a crystalline substance known as vanillin, can be obtained from coal-tar without the use of the plant. The researches of Tiemann and Haarman having shown that vanillin is a derivative of benzene containing the aldehyde group, one hydroxyl- and one methoxy-group, the synthesis of this compound soon followed (Ulrich, 1884). The starting-point in this synthesis is nitrobenzoic aldehyde, so that here again we begin with toluene as a raw material. A mixture of vanillin and benzoic aldehyde when attenuated to a state of extreme dilution in a spirituous solvent, gives the perfume known as “heliotrope.”
Not the least romantic chapter of coal-tar chemistry is this production of fragrant perfumes from the evil-smelling tar. Be it remembered that these products—which Nature elaborates by obscure physiological processes in the living plant—are no more contained in the tar than are the hundreds of colouring-matters which have been prepared from this same source. It is by chemical skill that these compounds have been built up from their elemental groups; and the artificial products, as in the case of indigo and alizarin, are chemically identical with those obtained from the plant. Among the late achievements in the synthesis of vegetable products from coal-tar compounds is that of juglone, a crystalline substance found in walnut-shell. It was shown by Bernthsen in 1884 that this compound was hydroxy-naphthaquinone, and in 1887 its synthesis from naphthalene was accomplished by this same chemist in conjunction with Dr. Semper.
Another recent development in the present branch of chemistry brings a coal-tar product into competition with sugar. In 1879 Dr. Fahlberg discovered a certain derivative of toluene which possessed an intensely sweet taste. By 1884 the manufacture of this product had been improved to a sufficient extent to enable it to be introduced into commerce as a flavouring material in cases where sweetness is wanted without the use of sugar, such as in the food of diabetic patients. Under the name of “saccharin,” Fahlberg thus gave to commerce a substance having more than three hundred times the sweetening power of cane-sugar—a substance not only possessed of an intense taste, but not acted upon by ferments, and possessing distinctly antiseptic properties. The future of coal-tar saccharin has yet to be developed; but its advantages are so numerous that it cannot fail to become sooner or later one of the most important of coal-tar products. In cases where sweetening is required without the possibility of the subsequent formation of alcohol by fermentation, saccharin has been used with great success, especially in the manufacture of aËrated waters. Its value in medicine has been recognized by its recent admission into the Pharmacopoeia.
The remarkable achievements of modern chemistry in connection with coal-tar products do not end with the formation of colouring-matters, medicines, and perfumes. The introduction of the beautiful dyes has had an influence in other directions, and has led to results quite unsuspected until the restless spirit of investigation opened out new fields for their application. A few of these secondary uses are sufficiently important to be chronicled here. In sanitary engineering, for example, the intense colouring power of fluoresceÏn is frequently made use of to test the soundness of drains, or to find out whether a well receives drainage from insanitary sources. In photography also coal-tar colouring-matters are playing an important part by virtue of a certain property which some of these compounds possess.
The ordinary photographic plate is, as is well known, much more sensitive to blue and violet than to yellow or red, so that in photographing coloured objects the picture gives a false impression of colour intensity, the violets and blues impressing themselves too strongly, and the yellows and reds too feebly. It was discovered by Dr. H. W. Vogel in 1873 that if the sensitive film is slightly tinted with certain colouring-matters, the sensitiveness for yellow and red can be much increased, so that the picture is a more natural representation of the object. Plates thus dyed are said to be “isochromatic” or “orthochromatic,” and by their use paintings or other coloured objects can be photographed with much better results than by the use of ordinary plates. The boon thus conferred upon photographic art is therefore to be attributed to coal-tar chemistry. Among the numerous colouring-matters which have been experimented with, the most effective special sensitizers are erythrosin, one of the phthaleÏns, quinoline red, a compound related to the same group, and cyanin, a fugitive blue colouring-matter obtained from quinoline in 1860 by Greville Williams.In yet another way has photography become indebted to the tar chemist. Two important developers now in common use are coal-tar products, viz. hydroquinone and eikonogen. The history of these compounds is worthy of narration as showing how a product when once given by chemistry to the world may become applicable in quite unexpected directions. Chloroform is a case in point. This compound was discovered by Liebig in 1831, but its use as an anÆsthetic did not come about till seventeen years after its discovery. It was Sir James Simpson who in 1848 first showed the value of chloroform in surgical operations. A similar story can be told with respect to these photographic developers.
Towards the middle of the last century a French chemist, the Count de la Garaye, noticed a crystalline substance deposited from the extract of Peruvian bark, then, as now, used in medicine. This substance was the lime salt of an acid to which Vauquelin in 1806 gave the name of quinic acid (acide quinique). In 1838 Woskresensky, by oxidizing quinic acid with sulphuric acid and oxide of manganese, obtained a crystalline substance which he called quinoyl. The name was changed to quinone by WÖhler, and, as we have already seen (p. 172), the term has now become generic, indicating a group of similarly constituted oxygen derivatives of hydrocarbons. Hydroquinone was obtained by Caventou and Pelletier by heating quinic acid, but these chemists did not recognize its true nature. It was the illustrious WÖhler who in 1844 first prepared the compound in a state of purity, and established its relationship to quinone. This relationship, as the name given by WÖhler indicates, is that of the nature of a hydrogenised quinone. The compound is readily prepared by the action of sulphurous acid or any other reducing agent on the quinone.
It has long been known in photography, that a developer must be of the nature of a reducing agent, either inorganic or organic, and many hydroxylic and amidic derivatives of hydrocarbons come under this category. Thus, pyrogallol, which has already been referred to as a trihydroxybenzene (p. 146), when dissolved in alkali rapidly absorbs oxygen—it is a strong reducing agent, and is thus of value as a developer. But although pyrogallol is a benzene derivative, and could if necessary be prepared synthetically, it can hardly be claimed as a tar product, as it is generally made from gallic acid. Now hydroquinone when dissolved in alkali also acts as a reducing agent, and in this we have the first application of a true coal-tar product as a photographic developer. Its use for this purpose was suggested by Captain Abney in 1880, and it was found to possess certain advantages which caused it to become generally adopted.
As soon as a practical use is found for a chemical product its manufacture follows as a matter of course. In the case of hydroquinone, the original source, quinic acid, was obviously out of question, for economical reasons. In 1877, however, Nietzki worked out a very good process for the preparation of quinone from aniline by oxidation with sulphuric acid and bichromate of soda in the cold. This placed the production of quinone on a manufacturing basis, so that when a demand for hydroquinone sprung up, the wants of the photographer were met by the technologist. Eikonogen is another organic reducing agent, discovered by the writer in 1880, and introduced as a developer by Dr. Andresen in 1889. It is an amido-derivative of a sulpho-acid of beta-naphthol, so that naphthalene is the generating hydrocarbon of this substance.
The thio-derivative of toluidine described as “primuline” (p. 160), has recently been found by its discoverer to possess a most remarkable property which enables this compound to be used for the photographic reproduction of designs in azo-colours. Diazotised primuline, as already explained, combines in the usual way with amines and phenols to form azo-dyes. Under the influence of light, however, the diazotised primuline is decomposed with the loss of nitrogen, and the formation of a product which does not possess the properties of a diazo-compound. The product of photochemical decomposition no longer forms azo-colours with amines or phenols. If, therefore, a fabric is dyed with primuline, then diazotised by immersion in a nitrite bath, and exposed under a photographic negative, those portions of the surface to which the light penetrates lose the power of giving a colour with amines or phenols. The design can thus be developed by dipping the fabric into a solution of naphthol, naphthylamine, &c. By this discovery another point of contact has been established between photography and coal-tar products. Nor is this the only instance of its kind, for it has also been observed that a diazo-sulpho-acid of one of the xylenes does not combine with phenols to form azo-dyes excepting under the influence of light. A fabric can therefore be impregnated with the mixture of diazo-sulpho-acid and naphthol, and exposed under a design, when the azo-colour is developed only on those portions of the surface which are acted upon by light.
The last indirect application of coal-tar colouring-matters to which attention must be called is one of great importance in biology. The use of these dyes as stains for sections of animal and vegetable tissue has long been familiar to microscopists. Owing to the different affinities of the various components of the tissue for the different colouring-matters, these components are capable of being differentiated and distinguished by microscopical analysis. Furthermore, the almost invisible organisms which in recent times have been shown to play such an important part in diseases, have in many cases a special affinity for particular colouring-matters, and their presence has been revealed by this means. The micro-organism of tubercle, for example, was in this way found by Koch to be readily stained by methylene blue, and its detection was thus rendered possible with certainty. Many of the dyes referred to in the previous pages have rendered service in a similar way. To the pure utilitarian such an application of coal-tar products will no doubt compensate for any defects which they may be supposed to possess from the Æsthetic point of view.[6]
From a small beginning there has thus developed in a period of five-and-thirty years an enormous industry, the actual value of which at the present time it is very difficult to estimate. We shall not be far out if we put down the value of the coal-tar colouring-matters produced annually in this country and on the Continent at £5,000,000 sterling. The products which half a century or so ago were made in the laboratory with great difficulty, and only in very small quantities, are now turned out by the hundredweight and the ton.[7] To achieve these results the most profound chemical knowledge has been combined with the highest technological skill. The outcome has been to place at the service of man, from the waste products of the gas-manufacturer, a series of colouring-matters which can compete with the natural dyes, and which in many cases have displaced the latter. From this source we have also been provided with explosives such as picric acid; with perfumes and flavouring materials like bitter-almond oil and vanillin; with a sweetening principle like saccharin—compared with which the product of the sugar-cane is but feeble; with dyes which tint the photographic film, and enable the most delicate gradations of shade to be reproduced; with developers such as hydroquinone and eikonogen; with disinfectants which contribute to the healthiness of our towns; with potent medicines which rival the natural alkaloÏds; and with stains which reveal the innermost structure of the tissues of living things, or which bring to light the hidden source of disease. Surely if ever a romance was woven out of prosaic material it has been this industrial development of modern chemistry.
But although the results are striking enough when thus summed up, and although the industrial importance of all this work will be conceded by those who have the welfare of the country in mind, the paths which the pioneers have had to beat out can unfortunately be followed but by the few. It is not given to our science to strike the public mind at once with the magnitude of its achievements, as is the case with the great works of the engineer. Nevertheless the scientific skill which enables a Forth Bridge to be constructed for the use of the travelling public of this age—marvellous as it may appear to the uninstructed—is equalled, if not surpassed, by the mastery of the intricate atomic groupings which has enabled the chemist to build up the colouring-matters of the madder and indigo plants.
A great industry needs no excuse for its existence provided that it supplies something of use to man, and finds employment for many hands. The coal-tar industry fulfils these conditions, as will be gathered from the foregoing pages. If any further justification is required from a more exalted standpoint than that of pure utilitarianism it can be supplied. It is well known to all who have traced the results of applying any scientific discovery to industrial purposes, that the practical application invariably reacts upon the pure science to the lasting benefit of both. In no department of applied science is this truth more forcibly illustrated than in the branch of technology of which I have here attempted to give a popular account. The pure theory of chemical structure—the guiding spirit of the modern science—has been advanced enormously by means of the materials supplied by and resulting from the coal-tar industry. The fundamental notion of the structure of the benzene molecule marks an epoch in the history of chemical theory of which the importance cannot be too highly estimated. This idea occurred, as by inspiration, to August KekulÉ of Bonn in the year 1865, and its introduction has been marked by a quarter century of activity in research such as the science of chemistry has never experienced at any previous period of its history. The theory of the atomic structure of the benzene molecule has been extended and applied to all analogous compounds, and it is in coal-tar that we have the most prolific source of the compounds of this class.
It was scarcely to be wondered at that an idea which has been so prolific as a stimulator of original investigation should have exerted a marked influence on the manufacture of tar-products. All the brilliant syntheses of colouring-matters effected of late years are living witnesses of the fertility of KekulÉ’s conception. In the spring of 1890 there was held in Berlin a jubilee meeting commemorating the twenty-fifth anniversary of the benzene theory. At that meeting the representative of the German coal-tar colour industry publicly declared that the prosperity of Germany in this branch of manufacture was primarily due to this theoretical notion. But if the development of the industry has been thus advanced by the theory, it is no less true that the latter has been helped forward by the industry.
The verification of a chemical theory necessitates investigations for which supplies of the requisite materials must be forthcoming. Inasmuch as the very materials wanted were separated from coal-tar and purified on a large scale for manufacturing purposes, the science was not long kept waiting. The laborious series of operations which the chemist working on a laboratory scale had to go through in order to obtain raw materials, could be dispensed with when products which were at one time regarded as rare curiosities became available by the hundredweight. It is perhaps not too much to say that the advancement of chemical theory in the direction started by KekulÉ has been accelerated by a century owing to the circumstance that coal-tar products have become the property of the technologist. In other words, we might have had to wait till 1965 to reach our present state of knowledge concerning the theory of benzenoid compounds if the coal-tar industry had not been in existence. And this is not the only way in which the industry has helped the science, for in the course of manufacture many new compounds and many new chemical transformations have been incidentally discovered, which have thrown great light on chemical theory. From the higher standpoint of pure science, the industry has therefore deservedly won a most exalted position.
With respect to the value of the coal-tar dyes as tinctorial agents, there is a certain amount of misconception which it is desirable to remove. There is a widely-spread idea that these colours are fugitive—that they rub off, that they fade on exposure to light, that they wash out, and, in short, that they are in every way inferior to the old wood or vegetable dyes. These charges are unfounded. One of the best refutations is, that two of the oldest and fastest of natural colouring-matters, viz. alizarin and indigo, are coal-tar products. There are some coal-tar dyes which are not fast to light, and there are many vegetable dyes which are equally fugitive. If there are natural colouring-matters which are fast and which are Æsthetically orthodox, these are rivalled by tar-products which fulfil the same conditions. Such dyes as aniline black, alizarin blue, anthracene brown, tartrazine, some of the azo-reds and naphthol green resist the influence of light as well as, if not better than, any natural colouring-matter. The artificial yellow dyes are as a whole faster than the natural yellows. There are at the present time some three hundred coal-tar colouring-matters made, and about one-tenth of that number of natural dyes are in use. Of the latter only ten—let us say 33 per cent.—are really fast. Of the artificial dyes, thirty are extremely fast, and thirty fast enough for all practical requirements, so that the fast natural colours have been largely outnumbered by the artificial ones. If Nature has been beaten, however, this has been rendered possible only by taking advantage of Nature’s own resources—by studying the chemical properties of atoms, and giving scope to the play of the internal forces which they inherently possess—
“Yet Nature is made better by no mean,
But Nature makes that mean: so, o’er that art,
Which, you say, adds to Nature, is an art
That Nature makes.”The story told in this chapter is chronologically summarized below—
1820. | Naphthalene discovered in coal-tar by Garden. |
1832. | Anthracene discovered in coal-tar by Dumas and Laurent. |
1834. | Phenol discovered in coal-tar by Runge. |
1842. | Picric acid prepared from phenol by Laurent; manufactured in Manchester in 1862. |
1845. | Benzidine discovered by Zinin. |
1859. | Corallin and aurin discovered by Kolbe and Schmitt and by Persoz; leading to manufacture from oxalic acid and phenol. |
1860. | Synthesis of salicylic acid by Kolbe. |
1864. | Manchester yellow discovered by Martius, leading to manufacture of alpha-naphthylamine and then to alpha-naphthol. |
1867. | Magdala red discovered by Schiendl. |
1868. | Synthesis of alizarin by Graebe and Liebermann, leading to the utilization of anthracene, caustic soda, potassium chlorate and bichromate, and calling into existence the manufacture of fuming sulphuric acid. |
1870. | GalleÏn, the first of the phthaleÏns, discovered by A. v. Baeyer, followed in 1871 by coeruleÏn, and in 1874 by the eosin dyes (Caro). These discoveries necessitated the manufacture of phthalic acid and resorcinol. |
1873. | Orthochromatic photography discovered by Vogel. |
1876. | Azo-dyes from the naphthols introduced by Roussin and Poirrier and Witt, leading to the manufacture of the naphthols, sulphanilic acid, &c. |
1877. | Preparation of quinone from aniline by Nietzki, utilized in photography in 1880 for manufacture of hydroquinone. |
1878. | Disulpho-acids of beta-naphthol introduced by Meister, Lucius, and BrÜning, leading to azo-dyes from aniline, toluidine, xylidine, and cumidine. |
1879. | Acid naphthol yellow introduced by Caro. |
" | Biebrich scarlet, the first secondary azo-colour, introduced by Nietzki. |
" | Nitroso-sulpho acid of beta-naphthol discovered by the writer; followed in 1883 by naphthol green (O. Hoffmann), and in 1889 by eikonogen (Andresen). |
" | Beta-naphthol violet, the first of the oxazines, discovered by the writer; followed in 1881 by gallocyanin. |
" | Coal-tar saccharin discovered by Fahlberg; manufacture made practicable in 1884. |
1880. | Synthesis of indigo by A. v. Baeyer. |
" | Quinoline synthesised by Skraup’s process. |
1881. | Kairine introduced by O. Fischer, the first artificial febrifuge. |
" | Indophenol discovered by KÖchlin and Witt. |
" | Azo-dyes from new sulpho-acid of beta-naphthol introduced by Bayer & Co. |
1883. | Antipyrine introduced by L. Knorr, leading to manufacture of phenylhydrazine. |
1884. | Congo red, the first secondary azo-colour from benzidine, introduced by BÖttiger. Beginning of manufacture of cotton azo-dyes, and leading to the production of benzidine and tolidine on a large scale. |
1885. | Secondary azo-dyes from benzidine and tolidine containing two dissimilar amines, phenols, &c., introduced by Pfaff. |
" | Tartrazine discovered by Ziegler; manufacture of sulpho-acid of phenylhydrazine and of dioxytartaric acid. |
1885. | Thiorubin introduced by Dahl & Co., leading to manufacture of thiotoluidine; followed by primuline, discovered by A. G. Green in 1887. |
1886. | Secondary azo-dyes of stilbene series introduced by Leonhardt & Co. |