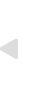
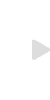
The Piston is perhaps the most important detail to consider, for it is on the piston that the force of the explosion acts when the heat energy is converted into mechanical energy. It must be made sufficiently strong to withstand the bursting effect of successive explosions, and yet if we make the metal too thick it will retain too much of the waste heat and the piston may seize in the cylinder due to expansion. To understand why the piston is likely to seize in the cylinder we have only to remember that when a metal body is heated it gets larger in every direction, but if cooled it returns to its original size. Now if we make the metal of the piston too thick so that the waste heat cannot pass quickly through it and dissipate itself at cooler parts of the engine, then the bulk of this heat will be concentrated in the piston head, causing it to expand and become a tight fit in the cylinder, as the cylinder walls are fairly thin and in contact with the jacket water which keeps them fairly cool and prevents them expanding much above their normal size. The actual amount of expansion is very small of course, but there is very little clearance between the piston and the cylinder walls, even when the engine is all cold—perhaps five-thousandths of an inch. The piston therefore must be strong, yet as light as we can make it, having regard to the necessity for its being amply stiff and rigid and able to stand up to its work.
Fig. 15.—Two views of a Cast Iron Piston with Gudgeon Pin and Packing Rings.
Generally it will be an iron casting in the form of a small cylinder (see Fig. 15), having provision in it for the packing rings P, and the gudgeon pin G, with its fastening screws S1, S2. The piston itself, as we have observed, must be a nice sliding fit in the bore of the cylinder without any shake or side play when there are no packing rings in the grooves. The packing rings are turned to size so as to fit the cylinder exactly and prevent any gas leaking past the piston into the crankchamber. These rings are very light, are made from cast iron, and arranged to break joint, as indicated, by cutting the middle ring in the opposite direction to the two outer ones. Bosses are cast on the inside of the piston and afterwards bored out to receive the steel gudgeon pin or wrist pin G. This pin is best made of plain parallel cylindrical form ground true, and the bosses in the piston should be reamered out to the exact size of the pin. When the pin has been inserted the tapered screws are screwed hard up by means of a special spanner and bear against the pin, preventing it from coming loose or from shaking or knocking. There are many other methods of fixing the gudgeon pin which are not shown here; each has some special point in its favour, but the one illustrated is undoubtedly the best and affords a positive adjustment for wear.
Fig. 16.—Method of fixing the Gudgeon
Pin which allows for Adjustment
after Wear.
An enlarged view of one of the bosses, showing the taper pin in detail and how the split pin Q prevents it from slacking back by contact with the wall of the piston, is shown in Fig. 16. Sometimes the lower part of the piston is made lighter by drilling holes through the walls. It is very important to reduce the weight of the piston as much as possible, otherwise the engine cannot attain a high speed, so that it becomes essential to bear this in mind when constructing engines for racing purposes. Frequently we find steel pistons used, as they may be made lighter for the same strength, and then steel piston rings may be used; they are not much in favour for ordinary motor-car engines because the steel pistons expand at a greater rate than the cast iron of the cylinder, so that there is more liability to seizure. The crown of the piston is sometimes curved upwards and at other times curved downwards, but more often it is flat as shown in Fig. 17. The gudgeon pin is sometimes made of mild steel, and the surface is then case-hardened in the centre where the connecting rod end bears. At the present time it is quite as common to find gudgeon pins made of special nickel steel or other steel alloys that do not require case-hardening. On the whole these special steels make the best gudgeon pins and stand the hardest wear.
Fig. 17.—Three forms of Piston Head.
Fig. 18.—Connecting Rod in the form of a Phosphor Bronze Stamping.
The Connecting Rod is another very important detail of the engine mechanism, its function being to transmit the force of the explosion from the piston to the crankshaft.
Fig. 19.—Steel Connecting
Rod turned out of the Solid Bar.
One end of the connecting rod moves up and down with the piston and oscillates (or swings to and fro) on the gudgeon pin, while the other end of the connecting rod travels in a circle, being pivoted at the crankpin and rotating in a circle which has for its centre the centre line of the engine crankshaft. This is clearly indicated in Fig. 18. On the suction stroke of the engine the piston has to be pulled down, as we have already seen; on the explosion stroke the greatest pressure acts on the piston and pushes the connecting rod down. Thus sometimes the connecting rod is being pulled and at other times it is being pushed; in each case it has to overcome the resistance of the engine and drive the car. It is evident, therefore, that the character of the load carried by a connecting rod is just about as complex and dangerous as it is possible for a system of loading to be, and great care has to be taken in the design of such rods to ensure adequate strength without undue weight, as this would tend to keep down the maximum speed of the engine. Another important consideration is the cost of production, and for this reason one often finds it in the form of a phosphor bronze stamping of I section, although the ideal form is a round section of steel with a straight taper from gudgeon pin to crankpin end, and having a hole bored right up the centre to reduce the weight without sacrificing much strength. When the rod is made in the form of a stamping between dies it is possible to turn out great quantities at very low cost and at a very rapid rate, whereas the round steel rods would require to be machined from the solid bar to compete in price with the others. When phosphor bronze is used it is only necessary to bore out carefully and face the bearings at the two ends for the gudgeon pin and crankpin; the bearing at the crankpin end is always formed with a removable cap to facilitate fitting it nicely to the crankpin, journal and also to allow for adjustment as the bearing wears. With steel rods it is necessary to cast a white-metal lining in the crankpin end and then bore it out to form the bearing, but the crosshead bearing is usually formed by a phosphor bronze bush. It is evident, therefore, that the steel rods are more expensive, but they make a splendid mechanical job. A steel connecting rod is shown complete in Fig. 19. Stamped steel rods of I section are also commonly used and are much better and stronger than those made entirely of phosphor bronze.
Fig. 20.—Crankpin and Crankwebs.
Fig. 21.—Four-throw
Crankshaft
The Crankshaft, as its name implies, is a shaft with one or more cranks or right-angled bends in it. Its function is to convert the sliding motion of the piston into the rotary motion of the flywheel and revolving shaft. A crankshaft with a single throw (or single crank) is shown in Fig. 20; a four-throw crankshaft is shown in Fig. 21; and Fig. 22 shows how an equivalent motion can be obtained by a single pin fixed into the face of a flywheel. This device (Fig. 22) is frequently used for motor-cycle engines. Crankshafts are always made of steel; sometimes mild steel is used, but more usually special alloys of steel containing chrome, nickel, vanadium, etc., are used. The general practice at the present time is to machine the crankshaft direct out of a solid bar of steel; this requires special jigs for holding the work and special tools for cutting the metal, but is the quickest, cheapest, and most satisfactory method to adopt. A few firms specialize in this class of work with high-grade steel and can supply crankshafts from stock.
Fig. 22.—Motor-Cycle Crankpin
fixed into the Flywheel.
It is easily seen by examining Fig. 18 that the crankshaft is being twisted in overcoming the engine resistance, while Fig. 20 shows that the crankshaft is being bent under the push from the connecting rod, so that we say the material of a crankshaft is subjected to combined bending and twisting, and as such a combination is not easy to resist we see now why special steel alloys are required for safety, combined with economy in material and reduction of weight. In Fig. 20 the crankpin is shown at A, the crank cheeks or webs at B1, B2 and the crankshaft proper at C. The portions of the crankshaft C which work in the bearings D1, D2 are termed journals. A crankshaft must be very stiff and not bend or twist sensibly, otherwise the shaft will vibrate when the engine runs up to speed—which would be very undesirable. It must be perfectly true with all the bearings absolutely in line and the journals well bedded down in their respective brasses (or bearings), otherwise mechanical troubles will arise. Each crank with its crankpin and webs forms a lop-sided or unbalanced mass, so that either (1) each crank must have its own balance weight as in Fig. 23, or (2) special balancing masses must be fitted at each end of the crankshaft. A convenient method of balancing the crankshaft is to have a fan pulley at one end and the flywheel at the opposite end, so that by drilling holes in the faces of these discs an amount of metal may be removed from them sufficient to balance the excess weight of the respective crankpins and webs. In Fig. 24 the shaded area indicates that portion of the crank which constitutes an unbalanced mass. Crankshafts for high-speed engines have always to be very carefully balanced, otherwise the engines will never run satisfactorily, the want of balance being greatly aggravated as the speed of rotation increases. Fig. 25 shows how the crankshaft of a two-cylinder engine may be balanced by drilling holes in the flywheel and fan pulley respectively, but the same effect may be produced by attaching balancing masses—this latter method would, however, be more inconvenient and expensive. The crankpins and journals are ground truly circular after being turned in the lathe as true as possible.
Fig. 23.—Sketch of a Balanced Crank.
Fig. 24.—Sketch showing
the unbalanced portion of
the Crank by Shaded Lines.
Fig. 25.—Two-throw Crankshaft balanced by
drilling Holes in Flywheel and Fan Pulley.
Fig. 26.—Sketch to illustrate the
Forces acting on a Flywheel Rim.
The Flywheel.—We have already described how the force driving the piston of a motor-car engine varies during the four strokes of the cycle, but we must note that it also varies considerably during each individual stroke. Thus, on what is known as the explosion stroke (or power stroke) of the cycle, the pressure at the commencement of this stroke may be exceedingly great and yet towards the end of the stroke the gases have expanded and the exhaust valve has been opened, so that the pressure acting on the piston is then very small. Again, on the compression, suction, and exhaust strokes, the piston has to be pushed or pulled by some means, as no power is being generated. Therefore, if the engine is to be self-acting and run continuously, some means must be provided for storing up the great force of the explosions and giving it out again on the idle strokes. The function of the flywheel is to store any energy given to it over and above that required to drive the car and to give it out again when required for performing the functions of compressing, exhausting, and sucking in gas, as well as to keep the car running steadily. It is simply a heavy wheel mounted on the end of the crankshaft which, when once started revolving at a high speed, is not easily stopped, and which will give up part of its energy each time its speed is reduced owing to the demands of the engine; but when the engine is generating power the wheel will speed up and store the excess—the mere fact that the wheel is heavy causes these changes in speed to occur slowly, and therefore on the whole the fluctuation of speed is not great when a suitable flywheel is fitted. The flywheel does not limit the maximum speed of the engine, as it could go on slowly increasing in speed if no resistance was encountered until the wheel finally burst or flew to pieces. Thus the flywheel does not regulate the speed of the engine; it merely smooths out the inequalities in the several strokes of the “cycle.” Flywheels of motor-car engines are now always made of steel, so that they can be safely run at speeds up to 3,000 revolutions per minute without fear of the rim bursting. All parts of the rim tend to fly off radially in the direction of the arrows as shown in Fig. 26 under the action of centrifugal force. A built-up flywheel is shown in Fig. 27, and one made from a single stamping of steel is shown in Fig. 28. Generally speaking, when a coned clutch is fitted one portion of it is formed on the inside of the flywheel rim as indicated in these two figures. When the construction shown in Fig. 28 is adopted the lining would be inserted after the clutch cone had been put into place; very often the lining is made up of sections which can be readily inserted or withdrawn after the cone is in position.
Fig. 27.—A Flywheel
built up from Steel Forgings.
Fig. 28.—A Flywheel
turned from a Steel Stamping.